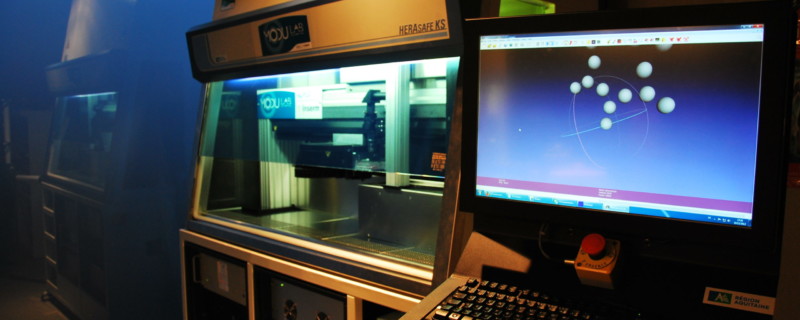
3D Bioprinting and 4D Bioprinting – Applications and Technologies
Introduction
3D bioprinting, a type of additive manufacturing, is the process of creating a three-dimensional (3D) structure by laying down successive sheets of living cells that build upon each other. The specific mechanical means of this deposition of cells and matrix vary greatly between bioprinters and bioprinting applications. The printing material used in bioprinting is often called “bio ink” and consists of living cells and material needed to support cell growth and proper tissue structure. The exact bio ink formulation varies depending on the cells being used and the structure being created.
The next generation of 3D bioprinting is called 4D bioprinting. A definition of 4D bioprinting was presented by Dr. William Whitford in last month’s “Another Perspective on 4D Bioprinting.” In the article Dr. Whitford explains that 4D bioprinting is “the printing of smart, environmentally responsive biological structures, tissues and organs. 4D bioprinting begins with the printing of multiple cells or biological matrices resulting in structures that undergo subsequent designed and anticipated (not spontaneous) but self-originated development in response to an environment.”
Dr. Whitford proposes the following comprehensive definition for 4D bioprinting:
The construction of individual multi-material printed objects from either living or preliminary substrates for medical or biotechnological applications when they are specifically engineered to respond in anticipated ways to user-demands or a changing environment by self-actuated shape-morphing, cellular differentiation, tissue patterning, defined biological characteristic alteration or functionality development.
It’s interesting to note that specifically what post-printing, engineered but self-actuated, construct changes can be included in the definition of a 4D bioprinted structure is still in debate.
3D Bioprinting and 4D Bioprinting Applications
There are several implementations of 3D/4D bioprinting applications that have tremendous potential to revolutionize healthcare. One example was published earlier this month in Nature Biotechnology. The article, “A 3D bioprinting system to produce human-scale tissue constructs with structural integrity,” was authored by a team of researchers at the Wake Forest Institute for Regenerative Medicine, led by Dr. Anthony Atala, Director of the Institute. In the article, authors describe how they were able to use a specialized 3D bioprinter to print ears, muscles and jawbones. In the abstract they state, “We present an integrated tissue–organ printer (ITOP) that can fabricate stable, human-scale tissue constructs of any shape.” This specialized system is able to print tissues containing microchannels, which serve to feed the cells until blood vessels can develop. This innovation presents a solution to the problem of how to get nutrients to cells that are in the inner core of the tissue until blood vessels develop. If these inner cells can’t get nutrients, they won’t survive and the tissue doesn’t become functional. The team used imaging data and computer modeling to control the printing of cells. They plan to use the ITOP to print tissues for use in human diagnostic and therapeutic applications and in the future more complex tissues and even solid organs.
To learn more about this application, please see the GeoBeats News Video below:
https://youtu.be/jvzu9MXC7e0
While most implementations are still in development phases, these and related technologies are now supporting some practical ADMETox modeling and have even had some clinical therapeutic successes with innovative institutions. See the BBC article –http://www.bbc.com/news/health-26885335
Full realization of the implications of this technology and others like it is still years from therapeutic use in humans, yet the current successes give us a glimpse into what is possible in the future with the use of 3D/4D bioprinting.
There is great interest in the ability to print replacement organs to replace traditional donor supplied organs, and with good reason. Everyday 22 Americans die while they wait for an organ to become available for transplant. However, most experts believe that this kind of advancement is at minimum, decades in the future. In addition to more accessible organs, there are significant advantages in using bioprinting for organ transplant. One of the primary being that the organs could be generated using a sample of the patients’ own cells, thus limiting or eliminating organ rejection issues and corresponding treatments that carry negative side-effects.
While these therapeutic applications often steal the headlines there are many other current or near term applications where 3D/4D bioprinting can make significant improvements in healthcare through drug discovery and drug testing. Using bioprinting, specially designed structures and tissues can be generated which provide assays and models for drug candidate screening, toxicity studies and disease modeling. Watch for a more detailed blog on these applications next month. Two companies working to develop these types of applications are Poietis and Organovo.
3D Bioprinting Partnerships
One approach to utilizing bioprinting technologies is to partner with a company that has developed their own bioprinting system and can partner to custom print different tissues. This allows companies to have access to printed tissues without having to possess the expertise, equipment, technology, etc. required to generate bioprinted tissues. Both Poietis and Organovo provide tissues, but not the bioprinters.
Poietis
One company that is available for partnering on custom printed tissues is Poietis. They successfully created bioprinted human skin models and last year signed an agreement with BASF to partner on research and development in cosmetics, specifically to improve BASF’s skin equivalent model Mimeskin™ and to test “advanced cosmetic bioactives for skin care applications,” according to a press release by BASF. One of the reasons BASF stated as a reason for wanting to work with Poietis is their laser-assisted 4D bioprinting approach.
I was fortunate to be able to talk with Mr. Bruno Brisson, Co-founder and Director of Business Development at Poietis about what makes their technology different from traditional extrusion based bioprinting. The primary difference comes from the use of a laser to assist in the bioprinting process. In their bioprinting process, a laser is focused on a cartridge of bio ink (living cells), and when the laser hits the cartridge microdroplets made up of a small number of cells all the way down to a single cell are deposited. Additional cells can be added and layered one upon another to create a very precise structure. The ability to deposit such a small number of cells permits very high resolution printing, around 15 microns. In addition, the cartridge is open and cells are not extruded, thereby reducing shear stress and ultimately leading to Poietis’ near 100% cell viability.
Mr. Brisson says that their business plan is to begin with the production of skin and other tissue models for cosmetic or pharmaceutical testing. The use of living tissue instead of animal models both improves biological relevance and removes ethical concerns surrounding the use of animals for testing. They also have plans to utilize their technology to custom print diseased tissues from the cells of patients. This permits screening of cancer therapeutics so that the best treatment can be selected for each patient – a personalized medicine approach. Printing tissues for therapeutic clinical applications is also in the plan, but that is in development and still several years down the road.
To support their custom tissue and therapeutic applications, Poietis has bioprinted over 25 different cell lines and has demonstrated that all are viable after printing.
In addition to the use of the laser, the development of their custom software was also key to their bioprinting tissue success. Poietis developed customized software to produce a blue print, which the bioprinter uses to control the exact position of cells and the printing of these cells in different layers. An important feature of this software allows the operator to try different configurations of cell structure and input the results. The incorporation of this trial and error learning enables constructive tweaks until functional tissue is produced. There was also significant effort placed in development of a cartridge that is properly matched with single cell accuracy and can accurately address number of cells deposited and viscosity of bio ink for each specific application.
While they don’t sell their laser-assisted bioprinters at this time, Poietis does contract with cosmetic and pharmaceutical companies who want to utilize their bioprinting technology to produce custom tissues. They welcome questions and requests for more information.
Video of Poietis’ laser-assisted bioprinting:
Organovo
Organovo, a San Diego based company, is currently using their NovoGen 3D bioprinting process to produce liver tissue for preclinical drug screening and toxicity testing. The process, described on their website, begins with a design of the tissue to be made. Then they use bio ink, “multi-cellular building blocks used to build target tissue,” released from a bioprinter in a layer by layer building approach. In November 2014, they launched their exVive3D™ Human Liver Tissue for preclinical drug discovery testing. This product allows companies to test drug candidates to discover potential liver toxicity and also ADME (adsorption, distribution, metabolism and excretion) outcomes.
According to their press release, the product is created using primary human hepatocytes, stellate, and endothelial cell types─ all found in the human liver. The tissues are functionally stable for at least 42 days. This is important for pharma companies who would like to be able to use liver tissue for drug challenges and need to be able to sequentially test various doses in the same tissue over a period of time.
In April 2015, Organovo presented data on their in vitro 3D kidney tissue at the 2015 Experimental Biology conference. This provides another tool for drug discovery and development, in particular examining a drug’s toxicity to the kidneys. The printed kidney tissue is “multi-cellular and fully human, consisting of polarized renal proximal tubular epithelial cells and a living interstitial layer comprised of renal fibroblasts and endothelial cells.”
In May 2015, Loreal and Organovo announced a partnership to utilize Organovo’s Bioprinting platform to create 3D printed skin tissue for Loreal’s product development and research. For over 30 years Loreal has been producing skin as a way to test products without having to use animal testing. It has lab facilities in France, where they grow skin samples from cells donated by plastic surgery patients. The partnership with Organovo, should enable more effective production of the skin model samples they need. The partnership is covered in more detail in the Bloomberg article, “L’Oreal’s Plan to Start 3D Printing Human Skin,”
In the future there is also the possibility that Organovo’s platform could be used to bioprint tissue for therapeutic applications.
3D Bioprinters
There are several bioprinters on the market to support the application of both 3D and 4D bioprinting. Instead of partnering to obtain tissue, a lab can purchase a bioprinter and print tissues directly. I have highlighted a couple 3D bioprinters and their associated technologies below.
Advanced Solutions’ BioAssemblyBot
One 3D bioprinter on the market with advanced printing capabilities is Advanced Solutions’ BioAssemblyBot. The BioAssemblyBot was the first to incorporate a robotic human-like arm into its 3D bioprinter. The use of the 6-axis robotic arm permits expanded capability in having the capacity to access any part of the printing surface, as well as its ability to move and flow just like a human arm. It really needs to be viewed, in action, to fully appreciate the function of the robotic arm (please see videos below). In addition to the robotic arm, Advanced Solutions has developed proprietary software called TSIM. TSIM can import a patient’s medical images, then once imported, these images can be isolated into a 3D model of specific tissue structures. These models serve as the basis for creating digital prototypes. The BioAssemblyBot then uses the TSIM model as the blue print for the 3D bioprinting.
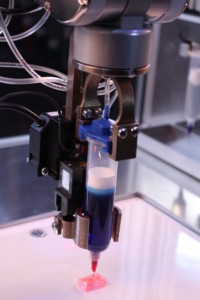
I was able to talk with Mr. Michael Golway, President and CEO of Advanced Solutions, about what made the BioAssemblyBot unique. I was impressed to learn that the development of the BioAssemblyBot, was the result of years of collaborative work between engineers and biologists. Mr. Golway said, “It was important to understand the biologist’s workflow and their bottlenecks, if we were going to create a 3D bioprinter to address all their needs.” The key feature is the robotic arm, which enables expanded printing capability and also experiment options. For instance, if a particular tissue requires a resting or incubation period between printing cell layers, the robotic arm could print the first layer, pick up the structure and place it in an incubator, then after the appropriate amount of time could retrieve the structure and print additional layers. In another example, the BioAssemblyBot can be loaded with different bio inks and needle sizes, based on the requirements of the 3D model. The robotic arm can change out the various bio inks and thus can print different cell types on the same structure. The use of the robotic arm allows for precise handling and the ability to incorporate multiple operating variables, all without the risk of human operator error or contamination. This feature further enables 4D bioprinting, which requires the incorporation of time for cells to grow, interact, and respond to various stimulus, etc., before the tissue is functional. It is impressive that this 3D bioprinter incorporates a combination of over 60 years of robotics understanding, coupled with an over a 30 year history of 3D printers to make a very sophisticated 3D bioprinter.
To see the BioAssemblyBot in action, please watch:
3D Printing Tissue Models in a 12 Well Plate
Printing the Heart – TSIM and BioAssemblyBot Workflow
Izumi International, Inc.
Another company that provides 3D printing solutions is Izumi International, Inc. Izumi designs and builds customized laboratory automation and bioprinting solutions for clients all over the world. They have an extensive portfolio of dispensing technologies, which they use to create customized solutions for applications where an off-the shelf dispenser isn’t successful.
Izumi’s bioprinters have enabled research in various laboratories, such as is featured in this Reuters video (with accompanying peer-reviewed publication of research performed on the Palmetto Printer), this TED Talk, and this Fortune article that highlights exciting results released just last week. These examples showcase 4D bioprinting, as the printer is merely a tool used to deposit patterns, and the cells themselves have shown the ability to self-organize and fuse into viable tissue.
Although bioprinting has remained in the realm of R&D for some time, it has recently begun to see adoption into more immediate, widespread, and feasible applications. For example, Izumi has been working with some of the top names in the pharmaceutical industry to optimize their drug discovery and incorporate bioprinted assays and models into their workflows. This will ultimately contribute to a much more streamlined clinical trial landscape, as the data generated from 3D assays vastly outperforms that from traditional 2D workflows. To meet the unique demands of well-plate formats, Izumi will soon be releasing a new bioprinter that can print into any well pattern with a noncontact jet dispenser. This bioprinter will also allow R&D users to create 3D scaffolds with an extrusion nozzle and seed cells using the jet printer.
Other bioprinter manufacturers include: RegenHu SA, Bio3D Technologies, 3Dynamic Systems, GeSiM, CELLINK, BioBots, and EnvisionTec.
For more reading on 3D Bioprinting and 4D Bioprinting, please see:
Another Perspective on 4D Bioprinting
3D Bioprinting – Research Successes, Challenges, and Future Possibilities
3D Printing and 3D Bioprinting – Changing the Healthcare Landscape
If you are interested in 3D and 4D bioprinting, don’t miss next week’s Ask the Expert where Katie Golson, Business Development Manager and Application Engineer, Izumi International will be answering questions on 3D and 4D Bioprinting.