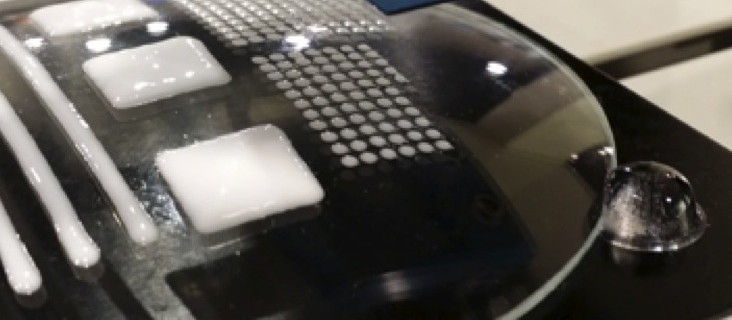
3D Bioprinting – Research Successes, Challenges, and Future Possibilities
A Guest Blog by Katie Golson, Biomedical Application Engineer, Izumi International
Background
In the United States, an average of 21 people die each day waiting for organ transplants. The need for organs far exceeds the number of available organs for transplant, and experts predict this donor shortage will only get worse as we continue to live longer:
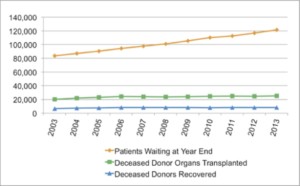
Based on data taken yearly since 1988, the OPTN (Organ Procurement and Transplantation Network) predicts the organ shortage will continue to rise.
What if a person waiting for a new donor organ, which might never be available, could somehow receive a genetically identical, healthy version of the diseased organ?
It might sound far-fetched, but this could potentially be possible in the future using 3D bioprinting technology. Theoretically, the patient’s own cells could be used to build a new three-dimensional replica of the faulty organ. There would be no need for anti-rejection medications and very little risk of rejection at all!
Bioprinting – an additive manufacturing approach to creating tissues in 3D (rather than the traditional 2D cell culture methodologies) – involves programming a robotic dispenser to deposit layer upon layer of biological and biocompatible materials (called “bioinks”) to create viable 3D tissue.
Though bioprinted organs like the one implied in Dr. Anthony Atala’s TED talk (“implied”, because it features not a living bioprinted organ but merely a suggestion of one) and even newly invented organs are (arguably) possible in the future, much of the current research focus is on smaller and more manageable pieces, like pharmaceutical testing tools. As with any industry in its infancy, standard practices are still evolving, and the hurdles faced in growing 3D tissues and organs are the subjects of exciting research all over the world.
This relatively new field has emerged from several established industries. Inkjet technology, optical and electronic applications, additive manufacturing or “3D printing”, and several biomedical applications have all influenced the formation of this niche. These are just a few of the continuously expanding examples, and 3D bioprinting will continue to evolve.
Though 3D bioprinting techniques are diversifying rapidly, inkjet printing has been and continues to be an important enabling technology for bioprinters. Originally conceptualized by Lord Rayleigh in 1878, the inkjet is a mechanism by which droplets of ink are propelled in a specified pattern onto a surface. The particular kind of inkjet technology with which we are now familiar (there are several types) was democratized almost a century later. Once this technology became affordable and ubiquitous in the late 1980s, research in its different applications, industrial and life science alike, began in earnest.
One such area has been in the field of tissue engineering/regenerative medicine (let’s consider them one field). A relatively established interdisciplinary field, the broad goal is to create functional tissues out of basic building blocks like cells, scaffolds, and various growth factors. Seeking new ways to create the 3D environment in which living cells can thrive, researchers have turned to bioprinting. The automation enables very high-resolution deposition of small droplets in 3D environments and is a promising area of focus in this and other biotech fields.
3D Bioprinting Successes
Though young, the 3D bioprinting field is entering a very exciting time. This technology and industry will see explosive growth, with the potential to disrupt many aspects of healthcare and drug development.
But when will this happen, and how far along are we?
A recent Forbes article, “No Donor Required: 5 Body Parts You Can Make With 3-D Printers,” outlined technical milestones in 3D bioprinting, which touches on the blood vessels, heart valve, skin, liver constructs, and ear. Most of these would require costly FDA approval in order to be considered for therapy and be commercialized. Luckily, some large companies are beginning to get in this game.
One company using 3D bioprinting to enable the drug development pipeline is Organovo in San Diego. Organovo announced last year it successfully delivered three-dimensional liver tissue constructs for experimentation. Their mission “is to build living human tissues that are proven to function like native tissues” to enable more physiologically relevant disease models, toxicology data, and potential regenerative therapies.
Just recently, L’Oreal and Organovo announced a partnership for 3D printed skin for cosmetics testing. Rather than use animals for clinical trials, L’Oreal has long been exploring the use of engineered skin for testing. They even sell this skin for “reliable and relevant in vitro tools for safety and efficacy profiling”, according to the Episkin website. They will explore the use of bioprinted skin for these tests as well.
Another recent headline features a Proctor and Gamble announcement for a grant competition for potential P&G bioprinting applications. As Elena Lurie-Luke, head of P&G’s Global Life Sciences Open Innovation, says: “If companies are doing innovation and interested in new tools, then bioprinting should very much be on their horizon.”
With the field starting to take off, one might wonder: Will bioprinting one day become a household task, wherein dressing a wound means applying one’s own cells back onto damaged tissue?
That is the idea behind the Armed Forces Institute of Regenerative Medicine (AFIRM)’s research into 3D bioprinting– that we can extract some healthy cells from a soldier, scan his wound to get 3D medical scans (CT scans, for example), create 3D mapping files, and use these files to direct the placement of the cells onto the wound to promote healing, rather than scarring.
While much exciting work is currently being done across various applications, many scientific challenges still lie ahead. As a biomedical application engineer, I am in a unique position to work with many researchers who are employing bioprinting as a way to address some of our most pressing healthcare needs. This means I also see first-hand the biggest challenges in bringing these therapies to reality.
3D Bioprinting Opportunity and Challenges
Viable three-dimensional tissue requires an efficient means of delivering nutrients and oxygen to its innermost cells. In the creation of tissue in the lab, vascularization remains challenging. One possible answer to this is to employ the biology of the cells themselves. This is what we have seen with our Palmetto Printer in use at MUSC. Using knowledge of muscle architecture and components, researchers have shown that they can program our bioprinter to deposit the biological components accurately enough to allow them to naturally fuse into vascularized tissue that can be implanted.
When building layer upon layer of cells to create 3D tissue constructs, structural integrity within the bioprinted sample is another challenging problem. Some researchers have designed strength into their system using a familiar 3D printing extrusion step. They use a thermosetting plastic to create the supporting lattice (an example is in the same TED talk mentioned above). Others are developing special bioinks that offer mechanical stiffness once deposited. Many of these require heat to achieve the lower viscosity required in some types of dispensing, which could damage cells. Alternatively, some methods of achieving a supportive framework require a finishing step to increase the stability once deposited; crosslinking a hydrogel is an example of this step. Finally, a simple method giving strength to the structure is to use additives that provide this structural support, and I will highlight one example using nanofiber in the next section.
In these examples, creating the 3D structural framework that will allow cells to survive for long periods of time (to enable laboratory research for now, and ultimately for translational purposes) is a problem of materials. Finding (or inventing) bioinks that are able to be dispensed, that will remain stable when printed, that are biocompatible and nontoxic, and that will also be degraded over time as the healing process takes over, is no small task. This will be the most challenging and crucial area of research moving forward.
Addressing Challenges: 3D Printing Nanofiber System
Lack of structural strength within the layers of bioprinted constructs is something we must address with our hardware as well as the bioinks we choose. At Izumi, we know that several different dispensing technologies will be needed in any good bioprinting system, giving researchers the capabilities needed for different applications. Because this field is so young, much of our effort in developing our platform focuses on finding appropriate 3D-printable biocompatible inks that work with our various dispensers. One particular material we are currently validating for our systems uses nanofibers.
An article published in Nanomedicine summarizes that “integrating nanofiber features is particularly important for recapitulating the Extracellular Matrix (ECM) architecture in the design and fabrication of scaffolds that host multiple cell types, and precisely define cell–cell and cell–matrix interactions in a 3D environment. Thus, nanofiber materials play a paramount role in tissue repair and regeneration. The development of nanotechnology allows for the fabrication of nanofiber scaffolds that are characterized by a nanoscale diameter, high surface area:volume ratio and high porosity.”
Those familiar with scaffolds used in tissue engineering might have experience with the electrospinning and meltblowing processes for creating nanofiber mesh. As an alternative, we recently developed through collaboration with Xanofi the world’s first 3D printing nanofiber system. Xanofi makes staple, discrete (short) fibers with length range of 100nm – 2um, a diameter of 500 nm, for an aspect ratio of 1:120. This unusually high surface area gives cells a lot of room, literally, for interaction and adhesion. The surface area of these nanofibers compared to electrospun or nonwovens is shown in this graphic:
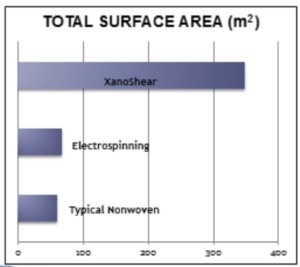
The Xanofi nanofibers have been shown to strengthen the ink, and the production process allows for customization of the chemistries; integration of functional groups and other particles or coatings is possible. We are currently validating the printer and ink combination and will offer it as a package, with the hope that researchers can spend less effort developing their own ink and more in running their experiments and advancing the field further.
Addressing Challenges: Non-Contact Pneumatically-Driven Mechanical Jet Dispenser
Another area of investigation at Izumi is the use of the Aerojet, a noncontact pneumatically-driven mechanical jet dispenser similar to inkjet printing but able to handle higher viscosities. This allows higher throughput, resolution, and ease of use to the bioprinting process.
Anyone who’s ever used a pipette or syringe knows that relying on contact and surface tension to deposit fluids can be frustrating, and that the substrate can be damaged by this contact. The jet mechanism avoids this frustration.
Fortunately, the Aerojet passes live/dead tests and precludes the need to factor in a Z-dimension for each dispensing action (though that is also possible, of course).
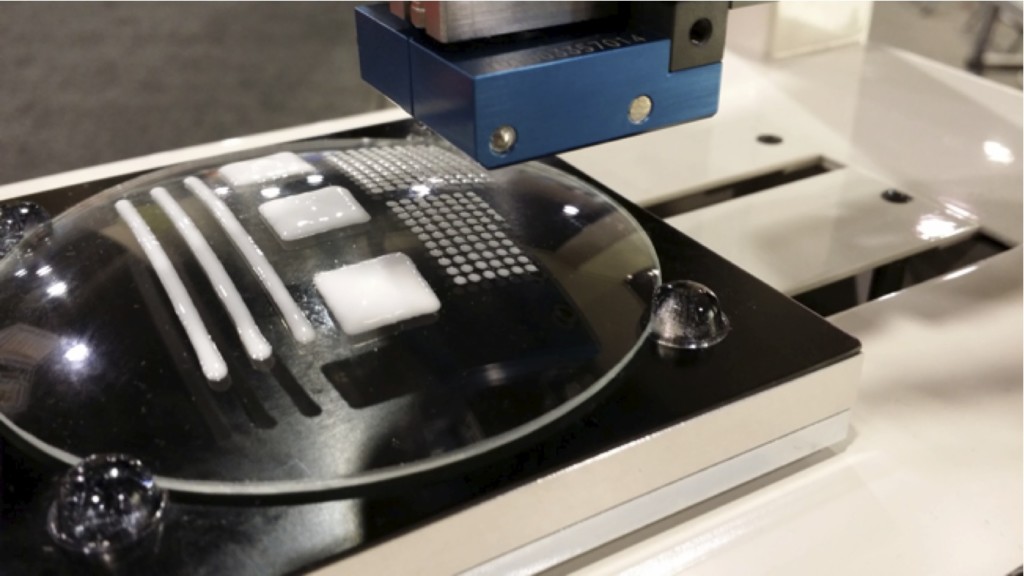
For labs seeding cells onto a 3D printed PLA ear structure, for example, the shape of the scaffold will no longer affect dispensing accuracy or speed (if the slopes are exceedingly steep, we can just use an articulating dispensing head to better address the angled surface). This is an area in which we have seen high interest because dispensing technologies struggle to successfully deposit live cells onto the scaffold, and they do so quite slowly in comparison.
We are actively investigating new polymers/scaffolds/biomaterials for dispensing, so if you have one you’d like to try, please contact me using information below.
Summary
Although there currently are some shortcomings and technical obstacles, the bioprinting market is expanding at a very rapid pace. New discoveries and advances are constantly pushing the field forward. The future of this technology depends on innovative new materials and ways to create more accurate physiological conditions, and we are working on finding solutions to those challenges!
Have you switched any of your processes to a 3D model? Do you think bioprinting has a place in your lab? Please share your thoughts in our comments section.
If you are interested in this field and want to read about more updates from Izumi, or you want to share some innovations going on in your lab, please feel free to connect with me on LinkedIn, twitter, or www.katiegolson.com (which I try to update with things we are working on regularly). You can also contact me directly at ktgolson@gmail.com.
Thank you.