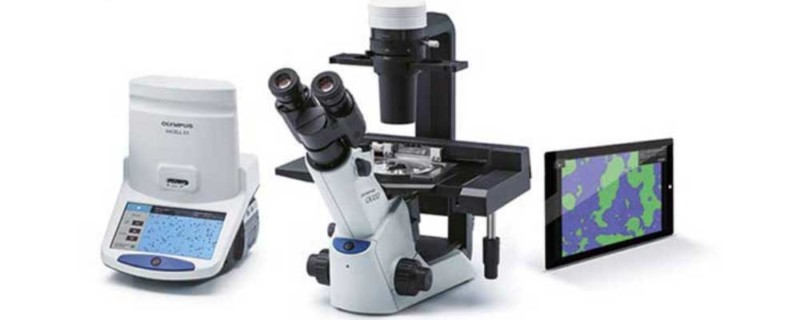
Accelerating Experimental Success Through a Systematic Cell Culture Workflow
The expansion and modernization of the cell culture process has sparked a demand for technologies that enable standardization and accurate documentation, while also increasing speed and reducing workload. This white paper explains the areas in which the latest technologies support the complete cell culture workflow, combining cutting edge optical and digital expertise to drive the future of cell-based applications.
An effective cell culture process lays the foundation for success in many application areas throughout life science and the pharmaceutical industry, from stem cell and cancer research to regenerative medicine. In order to ensure quality and reproducibility, the ability to clearly observe and document cells as they grow, proliferate and differentiate ex-vivo is central to following the progress of each culture at every stage of the workflow (Figure 1). Cell biologists must consider many fundamental aspects, and while how biologists manage each task often varies, modern laboratories are introducing approaches that ensure standardization and accurate documentation, alongside speed and efficiency.
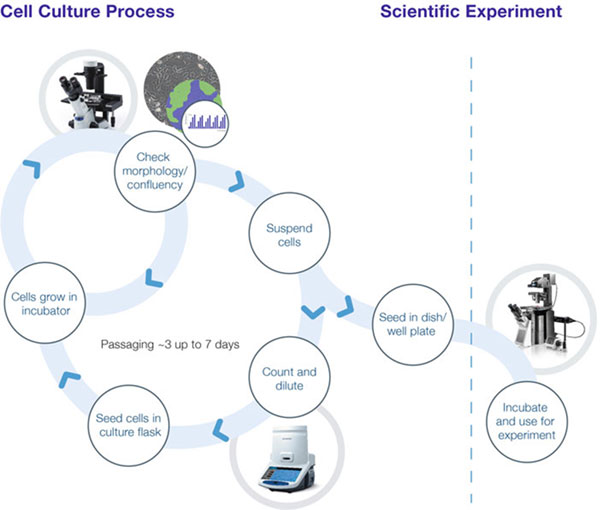
Once cells are plated, health and confluency are monitored under the microscope until they are dissociated and counted in-solution, for passaging, storage or experimental use.
- Standardizing conditions enhances experimental success, ensuring that samples are comparable. When cells are cultured in variable conditions, this alters growth patterns and in turn is highly likely to alter the gene expression of many cellular functions.
- Documenting results provides an accurate picture of cell behavior over time, allowing traceability for future reference, audits, peer review queries or patent applications.
- Speed and efficiency maintains culture health by minimizing the time cells remain outside optimum incubation conditions; while fast operation also frees up time for scientists to focus on other tasks.
These aims are facilitated by a range of highly optimized tools – from automated cell counters and software to specialized cell culture microscopes. Together these support a successful cell culture process that improves the reliability, reproducibility and ultimately the credibility of downstream experiments.
Enhancing Biological Insights
Success in cell cultivation arises from insightful observation and workflow efficiency. However, traditional light microscopy systems have limitations in terms of ease of use and ergonomics, leading to a time-consuming and uncomfortable workflow that can also compromise cell culture observation. As microscopy has evolved over the years, systems specialized for cell culture observation and analysis have incorporated a number of features to overcome these challenges (Figure 2). These include comfortable and convenient operation, which improve speed and overall efficiency. For example, a small and light UV-resistant system that fits inside the clean bench ensures all observations can be carried out under a sterile environment, reducing the risk of contamination. Ergonomic operation such as natural hand positioning for focusing and sampling also increases the speed of cell processing, which benefits the operator and the cell cultures themselves. Any additional time cells spend outside of optimum incubation conditions increases contamination risk and can cause stress, which can alter physiology and lead to inconsistencies between samples. In addition to operational considerations, the optical method employed also dictates the efficiency of the overall process.
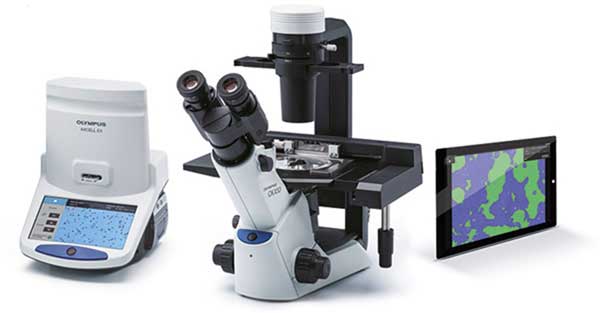
Standardization, efficiency and documentation a e enabled by Olympus cell culture solutions, including the CKX53 cell culture microscope, CKX-CCSW cell
Next Generation Observation
With the latest optics, cell culture microscopes facilitate fast and efficient screening, featuring a large field number to expand the field of view. With a field number of 22 producing images 21% larger than a field number of 20, this provides a more representative and clear sample overview, especially when using a 2X objective. Through an expanded field of view, this allows easy inspection of multiwell plates, which can now be observed in a single image.
In terms of the observation technique itself, standard light microscopes rely on brightfield imaging. However, this technique has one significant limitation making it unsuitable for cell culture applications in many cases. Known as phase objects, live, unstained cells such as those in culture do not absorb light, and many structures are therefore invisible under standard brightfield microscopy. Still, when light passes through these transparent samples, it does undergo a phase shift, and specialized illumination methods – including Phase Contrast [1] and the Hoffman modulation contrast method [2] – are capable of transforming phase shifts into a light intensity pattern to enhance image contrast. Phase Contrast is therefore by far the most popular method for cell biologists, but preparing and centering the different objectives is time consuming, especially when needing to switch magnifications. Allowing fast and high contrast observation of phase objects, a new integrated Phase Contrast (iPC) technology removes the need to individually change the ring slit when switching from 4X-40X objectives. As previously discussed, enabling a more efficient workflow can help maintain cellular health.
A New Phase in Stem Cell Microscopy
Although contrast imaging techniques are sufficient for standard cell culture inspection, this approach does have a limitation. Unfortunately, both Phase Contrast and the Hoffman modulation contrast methods fall short of achieving optimum image quality, giving rise to artefacts that interfere with the detailed observation of sample structures. While the application of Phase Contrast leads to a halo effect around the edge of the object obscuring the outline, the Hoffman modulation contrast method instead introduces a shadow in a direction determined by the equipment set up. The latest research efforts have focused on overcoming these limitations, and a new contrast technique known as inversion contrast (IVC) has been developed. As explained in Box 1, this novel method extends Phase Contrast illumination technology to create clear images with enhanced 3D information to deliver a greater level of optical information from a sample.
![]() |
IVC: Extending Phase ContrastIn the unique setup of IVC, both halos and directional shadows are removed, which is explained in greater detail in reference [3]. Essentially, an annular aperture is inserted into the light path at the front focal plane of the condenser (See image below). Since the aperture is larger than that used in standard Phase Contrast (the outer slit diameter is 10~20% larger than the pupil size and the inner slit diameter is 1~10% smaller than the pupil size), after passing through the sample, the beam illuminates the edge of the objective’s pupil. Therefore, only light passing through the sample at a small angle passes through the objective, whereas light at a large angle does not. When the sample has phase variation, the direction of the light passing through the sample changes. As a result, the image of the annular aperture is shifted slightly from the position of the pupil of the objective, and the area of the light flux passing th ough the objective increases. The increased light forms an image devoid of halos, and with the concentric arrangement of annular aperture and objective, the setup is independent of phase shift direction, eliminating directional shadows. The contrast is also inverted across the focal plane and the depth of field is educed, and the clear images with 3D information are ideal for analyzing iPS cell colonies. |
The IVC method significantly facilitates the morphologica analysis of 3D cells such as iPS cell colonies. Clearly observing iPS cell colonies, it is possible to detect changes in morphology suggesting cellular health or differentiation. Significantly, the IV method has been applied to visualize the outline and structure of mouse iPS cells (iPS-MEF-Ng-20D-17, Kyoto University [4]), capturing the 3D nature of the cells when compared to brightfield and phase contrast (Figure 3). Interestingly, IVC has been found to be ideal for distinguishing iPS cells from the surrounding feeder cells, as the contrast of the feeder cells is lower due to their flatter morphology highlighting the iPS cells against the background.
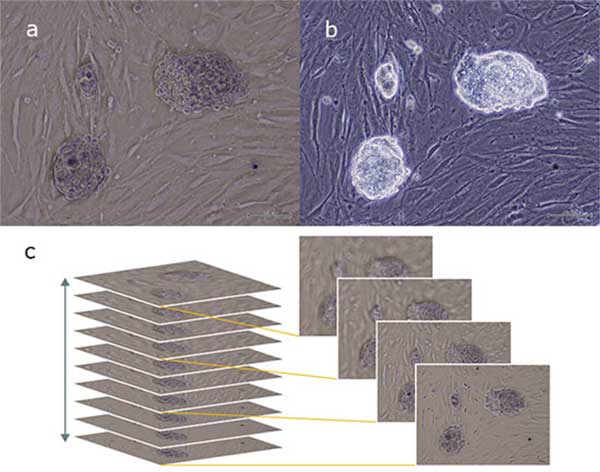
(a) in comparison with standard Phase Contrast (b). The new contrast technique provides enhanced 3D information (c).
Enabling Observation and Analysis
Another feature advantageous for cell cultivation is the ability to perform fluo escence imaging. Extending the utility of the cell culture microscope, fluo escence imaging capabilities allow the researcher to perform routine observation and functional studies on the same system, saving space and cost. A single system can be utilized for multiple stages of the cell culture workflow: f om monitoring the seeding, proliferation and passaging, through to high-contrast fluo escence imaging of cellular assays using a wide range of dyes.
Accurate Growth Monitoring
Observing cell culture in detail provides many important insights, for example whether cultures are differentiating and ready for further analysis. It is also recommended to observe cultures approximately every two days in order to closely monitor growth. Cell culture growth undergoes three distinct phases (Figure 4), and it is crucial to prevent the culture from proliferating beyond the log phase, where growth slows. In practical terms, the culture is ready to passage when confluency lies between 70-80%, and estimations a e often made visually, which can be highly variable. Advances in software technology have now introduced accuracy and standardization to confluency measu ements for adherent cells (Figure 2). Through employing such software with a cell culture microscope, quantifiable cell g owth data is quickly generated to ensure cells are always passaged at the correct time (Figure 5). Moreover, this enables scientists to create an accurate growth log, avoiding unnecessary dissociation and in-solution counting for the optimization of culture conditions, for example.
Cell Growth Phase
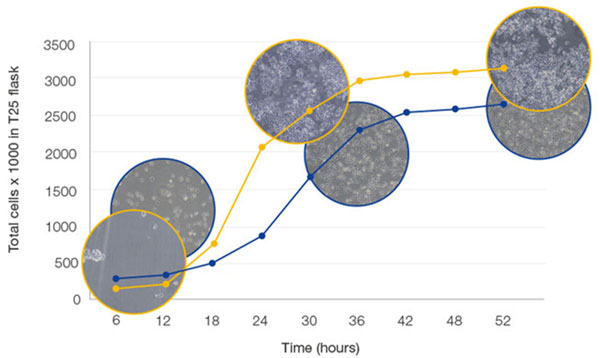
Cell proliferation begins in lag phase, turning into the exponential log phase as growth factor concentration increases. Growth plateaus as nutrients are consumed, and increased cell density leads to contact inhibition.
Monitoring Cell Growth
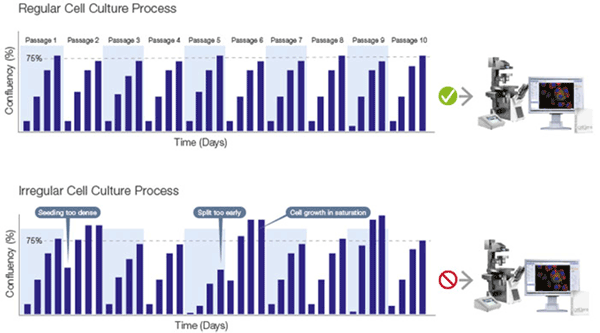
Choosing the right time to process proliferating cells is vital, ensuring they have grown enough for a sufficient yield, but have not yet reached saturation.
Systematic Processing
Whether preparing cell samples for passaging, downstream experimentation or storage, accurate in-solution cell counts are vital for the following reasons:
- Passaging – consistent seeding densities mean cultures grow at the same rate and health
- Downstream experimentation – identical cell counts facilitate comparable results
- Storing – knowing the cell concentration and viability in each vial is important when reviving cell line
Traditionally, this count is achieved manually with the hemocytometer. However, this slow and laborious technique introduces variations due to human error. A modern alternative is automated cell counting systems, which create an accurate cell count report in just 15 seconds that can be stored and exported, for streamlining, standardizing and documentation (Figure 2).
Summary
Cell culture forms the cornerstone of life science applications, and high quality results demand a high quality cell cultivation process. This can be enabled through considering many parameters and adopting the latest laboratory technologies available. Scientists are supported in perfecting a fast workflow that is full documented for future records, and highly standardized to achieve successful life science experiments and regenerative medicine applications.
About the Author
Jan Barghaan is the Strategic Marketing Manager Life Science EMEA, Olympus Europa SE & Co.
Footnotes
-
1. F. A. Ross, Phase Contrast and Interference Microscopy for Cell Biologists (Edward Arnold, 1967)
-
2. R. Hoffman, J. Microsc. 110, 205 (1977)
-
3. Suzuki, Y., Kajitani, K. and Ohde, H. (2015). Method for observing phase objects without halos or directional shadows. Optics letters; Vol. 40, No. 5. pp 812-815
-
4. K. Okita, T. Ichisaka, and S. Yamanaka, Nature 448, 313 (2007)