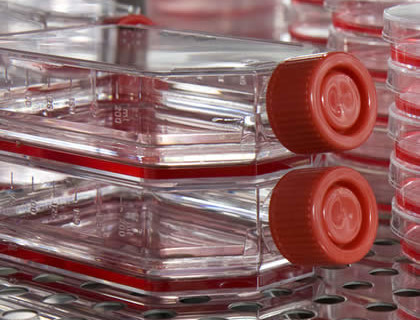
Achieving control and reproducibility of cell culture by eliminating serum
A guest blog by D.G. Ferneyhough Product Director, Cell-Ess serum replacement, Essential Pharmaceuticals
Scientists struggle to reproduce results from their own experiments or work from peer reviewed publications. There are many variables in play to develop representative models of tissues and organs. The choice of reagents including the use of fetal bovine serum (FBS) increases the variability and therefore complicates the design process and the interpretation of the results.
Several recent articles highlight the problems with reproducibility and the associated implications for advancing discovery work in basic research and drug discovery. Cells are incredibly sensitive to their environmental conditions including but not limited to cell signaling factors in media. Switching batches of serum or growth factors can make all the difference in the world (1). Elizabeth Iorns, co-founder of the Reproducibility Initiative stated, “The lack of reproducibility in cancer studies is a major obstacle in the development of viable therapies to cure cancer”. She went on further to imply the need to verify cancer studies and institutionalize scientific reproducibility (3). While serum has been empirically used in cell culture its relatively undefined nature is rarely questioned. FBS provides unknown but required factors for replication not present in basal media like DMEM. Even though FBS has been the reagent of choice to overcome the challenges of culturing primary cells it comes with a curse; in addition to the few required factors, a kitchen sink of other factors are also present. Surprisingly serum is thought of as one reagent and this has been shown to be the case. However each lot of serum is an independent reagent with different characteristics than another lot of serum. This can be demonstrated when one lot of serum can support the growth of cells and the associated functionality while another can not. This dichotomy accounts for a portion of the difficulty in reproducibility.
To reduce variability in experiments, researchers test different serum lots (2). Typically a lab might test 3 or more lots of serum to find one that works closest to the last successful lot used. Testing lots is thought of as a required chore, but is rarely put in a scientific context. To place it in stark terms, if the lab accepts 1 out of 3 lots, that represents a 67% failure rate, without questioning why there was high failure rate. If a 67% failure rate occurred with any other reagent there would likely be a systematic review of where and what caused the problem. It isn’t surprising that scientists have difficulty reproducing cell culture results intra-lab much less inter-lab, where it might be very difficult to acquire exactly the same lot of serum used.
What are the possible causes of variability in FBS lots?
Serum typically comes from pools of animals, fed a non-standardized diet, exposed to different environmental conditions and sacrificed at different times of year. It isn’t surprising that each lot is different. It is generally accepted that both genes and environment affect an organism. Thus, any individual cow is affected by both its DNA and its surrounding environment. While the discussion is centered on FBS, the mother, who is exposed to the environment, passes many factors to the fetus through the maternal blood. In terms of DNA, cows are heterogeneous and, unlike mice bred specifically for laboratory use, there are no genetically homogenized cow lines. Even if cows have identical DNA, the environment to which they are exposed can differ, including the types of microbes, weather, and diet, all of which have the potential to affect the health of the animal and, consequently, the particular myriad of elements contained in the blood. Since diet is not regulated among all cows, the levels of fats and sugars in the blood can be dramatically different from cow to cow.
However, given all of the possible variables experienced by each individual cow, the corresponding blood from each individual is also inconsistent, leading to lot-to-lot variability between each batch of serum isolated from that blood. Many of the factors that may affect the impact of serum on cells in culture are discussed in-depth below including proteins, lipids and hormones.
Depending on which of the many methods is used to measure the types of proteins in serum, studies show that serum contains anywhere from 200 to over 400 distinct proteins. The actual identity of the proteins can vary from lot to lot, as can the amount of each protein. The most abundant protein in serum is bovine serum albumin (BSA). It serves many functions, including binding free fatty acids and peptides. A range of different fats can exist in the blood depending on diet, meaning that different free fatty acids will be attached to BSA in serum harvested from different fetuses thus; the BSA protein itself presents an unknown profile in serum, varying from batch to batch. When serum is commonly used at a 10% concentration in culture, that 10% can be composed of different factors based on diet alone, and even differ from the same maternal cow during different times of the year. A whole host of other proteins are also present in serum at much lower quantities than BSA; including attachment factors, signaling factors, carriers, and transport proteins (4).
Proteins
Proteins in the form of attachment factors present in serum include, but are not limited to, fibronectin, vitronectin, collagen, and hyaluronic acid. Some of these attachment factors are present in serum, but not in the interstitial fluid normally surrounding organ cells in vivo; moreover, some attachment factors are present in clotted serum, but not in circulating blood. The presence of attachment factors has a variety of effects on different cell types. For example, binding to attachment factors can initiate an inflammatory response in antigen-presenting cells in the immune system, like macrophages and dendritic cells (5, 6). In stem cells, attachment factors can initiate differentiation (7, 8). In endothelial cells, attachment to a surface via an attachment factor can activate specific pathways depending on the particular attachment factor.
Proteins in the form of signaling molecules like growth factors are also present in serum and act as signaling factors. Interestingly, the particular level of a given growth factor can have implications on differentiation and activation of the cultured cell. As an example insulin-like growth factor (IGF) has recently received attention for having an effect on cells other than stimulating growth when present in high concentrations. Another signaling factor present in significant amounts in serum is transforming growth factor beta (TGF-B) (9), a potent signaling factor in cancer (where TGF-B suppresses immune responses, allowing the cancer to take hold), fibrosis (where TGF-B plays a role in disease progression), and in many other systems. Many other proteins in serum can have an effect on cultured cells. In some cases, the identity of those proteins is not known, while other proteins, like those involved in the complement cascade, are actively removed. Indeed, serum can be treated in various ways, like IgG removal or complement removal, in an attempt to remove their potential effects on cultured cells, but even removing those proteins may have other effects on cells that are not measured or accounted for in experiments.
Hormones
FBS typically originates from both sexes so it encompasses many hormones. The combination of testosterone and estrogen in a single batch of serum will be used here as an example to explain the ramifications of having hormones present in an uncontrolled and variable manner. The release of sex hormones is normally tightly regulated in vivo, and cell sensitivity to hormone levels is consequently also tightly regulated. Furthermore, cells used in an experiment typically originate from an animal of a single sex. As an example of a potential adverse consequence, exposing female-derived PBMCs to testosterone in cell culture will dramatically affect cell function. Besides naturally released hormones, hormone injections by humans can also introduce unknown hormones (of unknown quantities) into the animals depending on the country of origin or on the practices of a particular farm.
Lipids
Similar to the other constituents discussed above, lipids can also vary from lot to lot. As mentioned earlier BSA can carry lipids that participate in a wide range of activities. A recent paper showed that the lipid fraction in serum could signal dendritic cells (10). Indeed, lipids play an important role in cell membrane fluidity as well as in the membrane’s ability to respond to stimuli; moreover, lipids can sometimes act directly as signaling molecules. In cases where investigators are aware that lipids can affect their cultured cells, their attempts to remedy this by removing lipids via non-discriminate fat-removal methods (such as charcoal treatment) can also lead to unintended consequences on the cultured cells.
Conclusions
FBS has been used in cell culture for decades, and its use is actually increasing. However, in many cases, serum may not be the appropriate buffer or supplement to use in cell culture, as it does not represent physiological conditions and may alter the experimental output or assay. Furthermore, serum contains many factors that are not controlled, which can have a direct or indirect effect on the cells in culture. Lastly, even if factors are controlled for in one lot of serum, the probability of acquiring a similar lot for an experiment or project approaches zero due the combination of the changing environment and genetic variability in cows and their fetuses. Based on the variable and uncontrolled nature of FBS as detailed above, the use of serum in the biotechnology arena may be questioned for either developing cell therapeutics or generating protein products. First, the ability to control cellular output (as measured by protein production or cell functionality) is crucial for any FDA-approved or future FDA-approved therapy, and the use of serum presents obstacles to creating a reproducible product. Second, there may be issues related to FBS supply in terms of finding a source that can provide not only the required lot of serum but sufficient quantities of that particular lot for an entire project.
The variability and unknowns in serum also pose a challenge to its use in research. Indeed, the overall goal in research is to conduct a hypothesis-driven project in which results and interpretations depend on the ability to precisely control all variables and hold the remaining components of an experiment as constants. Moreover, reproducibility of experiments is essential, and the variability in serum may impede obtaining reproducible results independent of the actual biological system being investigated. Thus, the use of serum has many variables that cannot be controlled for and differ from lot to lot, therefore rendering the ability to follow the strict guidelines of hypothesis-driven work difficult.
One of the earliest attempts to remove serum from cell cultures was performed by Ham in the 1960s. While the idea of moving away from the use of serum has gained recognition and importance, developing a robust serum-free media has been difficult. Currently, a limited number of serum-free media specifically designed for a cell line is available. To develop such a specific media, a serial clonal selection (also known as adaptation) is performed for each cell line. While many serum free media have made advancements they still lack the physiologically important components missing from either serum or interstitial fluid namely lipids and cholesterol.
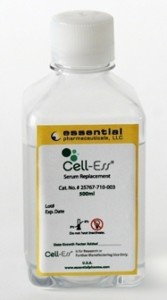
There is still an unmet need; consequently, researchers are learning how to build media that contain the necessary constituents for culturing cells, including amino acids, vitamins, lipids, salts, and growth factors. They want to achieve improved reproducibility and control over that of FBS in both the biotechnology arena and the academic research field.
Researchers are in a situation where they need to build media that don’t contain uncontrolled components like TGF-B, micro RNA, exosomes, or other components that obscure or impact a downstream bioassay. When building a chemically defined media, including essential fats is critical for cell synthesis and healthy cell culture. Cell-Ess® is an example of a synthetic media that works for many cell types, provides those essential fats in a novel, bioavailable manner, is a chemically defined, animal component free, that doesn’t change from lot to lot.
For More Information:
http://www.cell-ess.com/why-cell-ess/white-papers/
References
2) http://www.biocompare.com/Editorial-Articles/173903-Seeking-a-Happy-Cell-Culture-Medium/
4) Zheng, X. et al. Proteomic Analysis for the Assessment of Different Lots of Fetal Bovine Serum as a Raw Material for Cell Culture. Part IV. Application of Proteomics to the Manufacture of Biological Drugs. Biotechnology Progress 22, 12941300 (2008).
5) Sprague, L. et al. The interplay between surfaces and soluble factors define the immunologic and angiogenic properties of myeloid dendritic cells. BMC immunology 12, 35 (2011).
6) Acharya, A. P., Dolgova, N. V., Xia, C. Q., Clare-Salzler, M. J. & Keselowsky, B. G. Adhesive substrates modulate the activation and stimulatory capacity of non-obese diabetic mouse-derived dendritic cells. Acta biomaterialia 7, 180–92 (2011).
7) Linsley, C., Wu, B. & Tawil, B. The effect of fibrinogen, collagen type I, and fibronectin on mesenchymal stem cell growth and differentiation into osteoblasts. Tissue engineering. Part A 19, 1416–23 (2013).
8) Li, Y. et al. Differentiation of oligodendrocyte progenitor cells from human embryonic stem cells on vitronectin-derived synthetic peptide acrylate surface. Stem cells and development 22, 1497–505 (2013).
9) Dews, M. et al. Masking epistasis between MYC and TGF-β pathways in antiangiogenesis-mediated colon cancer suppression. Journal of the National Cancer Institute 106, dju043 (2014).
10) Leslie, D. et al. Serum lipids regulate dendritic cell CD1 expression and function. Immunology 125, 289–301 (2008)
11) Brunner, D. et al. Serum-free cell culture: the serum-free media interactive online database. ALTEX 27, 53–62 (2010).
12) Gilanyi, M., Fekete, J., Ikrenyi, C. & Kovach , G. B. Ion concentrations in subcutaneous interstitial fluid: measured versus expected values . Am J Physiol Renal Physiol 255, F513–F519 (1988).
13) Maggs, D. G. et al. Interstitial fluid concentrations of glycerol, glucose, and amino acids in human quadricep muscle and adipose tissue. Evidence for significant lipolysis in skeletal muscle. The Journal of clinical investigation 96, 370–7 (1995).
14) http://www.nature.com/nature/journal/v483/n7391/full/483531a.html