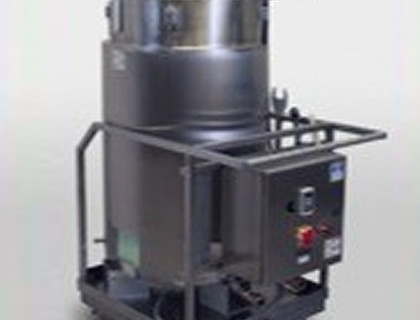
Advances in Adherent Cell Culture Approaches Abound – Promoting Progress in Production Performance for Attachment Dependant Processes
A Guest Blog by: William G. Whitford, Sr. Market Manager, Emerging Technologies, Thermo Scientific Cell Culture and BioProcessing
Large-scale adherent cell culture is required in such processes as vaccine manufacturing; cell-based therapies (including stem cell); cell replacement and tissue repair. The vaccine industry is completing its move from production in animal and primary tissues (such as embryonated chicken eggs) to cultured cell-based platforms. A number of adherent animal cell types are used by vaccine manufacturers, including mammalian and avian lines for both viral and the newer “viral-vectored” vaccines. Many animal and human vaccines are produced in attachment-dependent cells such as VERO, MDCK or chick embryo fibroblast (CEF) cells. Some of the established adherent lines have been used for decades in classic production formats. The viruses used for such work range from clones of influenza to recombinant lentivirus, baculovirus or alphavirus.
Traditional microcarrier-based culture
Microcarriers are one means of supporting the large-scale culture of “adherent” or “attachment-dependent” cells. They are small beads of glass, plastic or other composition having various densities, porosities and surface treatments that are dispersed in a suspension within a culture vessel. Upon these small beads certain cell types will adhere and proliferate. Unlike some adherent-culture supporting formats, microcarriers demonstrate extreme flexibility in the shape, size, charge of the matrix, as well as ease in accepting diverse types of derivatized surfaces.The industry typically refers to animal cells in culture as “adherent” or “suspension” culture, but a closer examination reveals a few interesting things about this distinction. The various types of cultured cells display differences, and almost a continuum, in their degrees of “attachment”. That is, some cells adhere to particular surfaces very tightly and can be difficult to remove. Other cells attach moderately well and are what we normally think of as in attached cell culture, and yet others do in fact adhere, but can be removed by simple physical agitation. Some cells constitutively require a solid support for culture, and other easily switch between adherent and suspension cultures. The reasons for this are complex, and are related to the many means by which cells attach. In brief, it should be understood that functionally, cell attachment is a dynamic interplay between the type of cells employed; the basic culture substrate and any surface modifications; and the culture media and supplements.
In any event, manufacturers must consider the individual adherence characteristics of not only the cell type they are employing, but of the particular construction or clone they have selected. They must also consider the properties of a final product as well as the surface of each culture method employed, including distinctions between methods employed in process development and manufacturing, and between seed expansion and final bioreactor culture. So, microcarriers are one of many approaches to supporting the large-scale culture of the more attachment-dependent cells. It should also be mentioned that they play an important role in such smaller-scale biopharmaceutical-related cell-culture applications as identifying and characterizing drug candidates.
Many cell-based therapeutics have been based upon attachment-dependent cells of endothelial, epithelial or mesodermal origin (including, eg, dermal fibroblasts) but lately cells of even embryonic endodermal origin have been used. These include such renowned types as adipocytes, chrondrocytes, pre-dendritic monocytes, macrophages as well as human pluri- or multi-potent stem cells (such as MSCs) and their differentiated derivatives. Cell mass expansion for generation of therapeutic quantities of these cells requires highly-controlled large-scale adherent culture in a clinical setting.
Methods of adherent cultures scale-up for production
Scale-up of cell mass for large-scale adherent cell-based manufacturing is of particular concern to product sponsors. As attachment-dependent culture processing generally requires more steps and time than suspension, there is a drive to design new approaches to reduce steps. Processes recently developed include cryopreserved working cell stocks of greater volume, supporting both larger initial culture and seeding at higher densities; intensified culture methods supporting higher peak culture densities (and therefore more seed to the next stage) as well as culture systems with a greater turn-down ratio allowing seeding of large-scale culture reactors with a lower cell mass. Here again, microcarriers present the greatest ease in format expansion for scale-up. It is true that scale-specific and ancillary issues do arise in tech–transfer, but unlike some other approaches the format itself does operate nominally through nearly any scale.
New developments in our understanding and control of cell attachment and detachment
There are three drivers of better-understanding the attachment process itself: 1) the development of new manufacturing formats and their materials, 2) the development of serum-free, protein-free and animal product-free media, and 3) the establishment of entirely new therapeutic approaches requiring new types of attachment-dependent cells. The academic sector is advancing rapidly in elucidating the many mechanisms of cell attachment such as the numerous glycoproteins directly involved, and transglutaminase as an integrin-binding adhesion co-receptor for fibronectin. This contributes to the ongoing development of two technologies promoting the binding of cells to a culture surface.
First, new biomimetic or other attachment-promoting surface chemistries such as charge and charge density generation, plasma treatments (and other energetic oxidations), covalently bound polyacrylamide and other moieties, electrospun microfibrous scaffold development, poly-x-lysine, synthetic hydrogels, and hydrophobicity modulation have been developed to address cell binding.Companies in this area have developed several different options when it comes to engineered surfaces and have applied these to a variety of cell culture products and systems. Some examples of innovative surface types include:
- Hydrophilic surfaces to bind water and repel cells
- Temperature-sensitive chemistry which binds cells then releases them at specific temperatures – no need for enzymes.
- Biomimetic surfaces employing, eg, synthetic peptide-based coatings to enhance attachment, proliferation or differentiation
- Specialized surfaces to mimic different in vivo environments for drug screening and cell function analysis.
Secondly, biologically-based surface coatings, matrices, and media additives that promote the attachment process have been developed. These include a variety of proteins and peptides, chondroitin sulfate, and other natural and synthetic extracellular matrices or matrix components such as hyaluronic acid, collagen, laminin, fibronectin (cold-insoluble globulin), vitronectin, gelatins, and reconstituted naturally secreted basement membrane mixtures. However, while these are often available as commercial preparations, there is usually an extended development term between successful research application and employment in biomanufacturing, as economical and robust processes here are often quite difficult to establish in large-scale. Note though, that some particular cells do adhere to appropriately derivatised cell culture surfaces rather easily– even in serum free media.
Similar research is contributing to our understanding of cell detachment. This and developments in novel surface chemistries has contributed to new synthetic, thermally- and chemically-directed cell release mechanisms. Such research has also determined developments in the traditional physical, chemical and enzymatic procedures to release cells. These traditional approaches include the use of physical shear, trypsin, hyaluronidase, collagenase, as well as other heterogenic or recombinant enzymes and enzyme mixtures against basement membrane components.
Developments in up- and down-stream manufacturing processes
Anchorage-dependent-cell cultivation formats include many “2D” planar approaches, such as roller bottles and multiple- or stacked-surface based systems of many types (including taunt gas-permeable films and so-called “hyper” technology). Beyond automating some operations and they can in some implementations even control such traditional culture parameters as dO2, dCO2, and pH. There are also a growing number of novel completely controlled so-called “3D” approaches including packed-bed bioreactors employing microfibers, hollow fibers and porous structures or hydrogels. All provide, to one degree or another, the two major advantages of a) higher cell density per unit volume than monolayer cultures and b) production in a single controlled bioreactor as opposed to manual handling of multiple smaller culture units such as roller bottles.
As introduced above, at this time microcarriers in stirred tank reactors provide the most popular platform for cell production by providing a large surface area for cell growth with ease of scale-up, reduced footprint, better process control, reproducibility, reliability, and a drastic reduction in labor.
Developments in microcarrier-based suspension platforms include single-use systems in stirred tank bioreactors and support of microcarriers in perfusion and intensified perfusion bioreactor culture. New microcarrier-supporting equipment provides process-ease and increased flexibility in production mode and format. For example, single-use systems such as SUBs are appearing with hydrodynamic styles that have been shown to be highly compatible with microcarrier-based manufacturing of even cells exhibiting a highly shear-sensitive phenotype. Intensified perfusion microcarrier culture is supported by devices, which not only support perfusion culture but new approaches to microcarrier separation at harvest. All-in-all, microcarriers have been reported to provide the best set of features for such large-scale manufacturing.
Other brand new approaches to the harvesting of microcarrier cultures are also appearing. Beyond the traditional gravitational settling, tangential flow filtration (TFF) and sequential differential centrifugation techniques have been employed to separate culture medium from microcarriers and cells. But they do require significant optimization and validation in large-scale manufacturing. Recently a novel single-use bag-in-bag method has appeared.
Developments in microcarriers themselves and their culture media
Recently there have been significant developments in many materials of composition and technology for attachment-dependent culture, including in microcarriers. New, low-density matrices that can be easily suspended in bioreactors come as solid or microporous substrates composed of diverse rigid or pliable materials. Microcarriers with ultra-low particulate level and/or bearing a designation of entirely animal product-free are now available for regulated pharmaceutical manufacturing.While most are spherical and smooth, others have macroporous surfaces or alternative dimensions such as rods or more planar hexagonal shapes. Additional advances include the infusion of magnetic particles that may help in cell separation. Gamma irradiated microcarriers are shipped in standards-compliant packaging greatly easing set-up.
The biopharmaceutical industry has been moving toward chemically defined and animal product free culture media for years, and this is true for attachment dependent cells as well. New media are appearing regularly claiming various regulatory status and performance for particular types of cells. While commonly providing new options for research and small-scale culture, for bioproduction such new media often disappoint because they either do not completely live up to the claimed properties, lack some of the ancillary properties required in large-scale manufacturing, or the small companies that have commercialized them do not understand the industry sufficiently to properly fully support such a product.
As the science of supporting the serum-free culture of adherent cells advances, sponsors of media formulations with the depth to fully support regulated manufacturing are launching incrementally superior formulations. At the research level, there are serum-free media formulations supporting stem cells and primary cells; eg, human embryonic and induced pluripotent stem cells under feeder-free condition. Some basal formulations should be used in conjunction growth or attachment factor supplements. How such formulation will perform on the manufacturing floor for the most part remains to be seen, because in many cases there exist additional media requirements imposed on the process by such diverse sources as a) large scale culture per se; b) the particular production format employed; c) the particular virus being cultured; d) the degree of product understanding and characterization accomplished to date; e) product-specific safety and regulatory constraints, and f) differential cell mass generation vs. viral product accumulation requirements. In any event, many premiere organizations are now involved in the development and commercialization of application-specific, inexpensive xeno-free GMP-compatible serum-free media for microcarrier-based culture.
Summary
When it comes to selecting a production method for adherent cells, the choices are numerous. Perhaps most important is finding the best production mode and culture format for your product and scale of manufacturing. Production methods vary and have strengths that are often best suited for specific cell lines, product types and scale. Then, comes the selection of a culture media that; a) robustly supports the cell type and clone being used; b) provides optimized production in the regulatory classification required; and c) is available from a dependable, compendia compliant manufacturer. Investigate these options and, when possible, ask for applications data on how the system performs with your cell and product type.
Author’s Bio:
William G. Whitford
Bill Whitford is Sr. Manager, Emerging Technologies for Thermo Scientific Cell Culture and Bioprocessing in Logan, UT with over 20 years experience in biotechnology product and process development. He joined the company nine years ago as a team leader in R&D developing products supporting biomass expansion, protein expression, and virus secretion in mammalian and invertebrate cell lines. Products he has commercialized include defined and animal product-free hybridoma media, fed-batch supplements, and aqueous lipid dispersions. He had previously served as Manufacturing Manager for PHASE-1 Molecular Toxicology in Santa Fe, New Mexico producing custom and standard DNA microarrays. An invited lecturer at international conferences, Bill has published over 150 articles, book chapters and patents in a number of fields in the bioproduction arena. He now enjoys serving on the Editorial Advisory Board for BioProcess International.
Author’s Particulars:
William G. Whitford Sr.
Market Manager, Emerging Technologies
Thermo Scientific Cell Culture & BioProcessing
Thermo Fisher Scientific
925 West 1800 South
Logan, Utah 84321