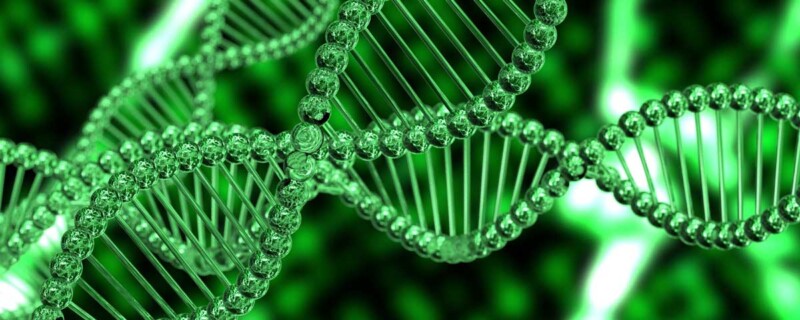
Advances in Sustainable Oligo Manufacturing
Synthetic oligonucleotides (ONs) are short pieces of nucleic acid being successfully applied for therapeutic purposes. Oligonucleotide therapeutics (OTs) have become established in diverse pipelines, entity types, and clinical trials. For example, they now include such unique structures as antisense RNAs, microRNA (MIR), small interfering RNA (siRNA), and aptamers. OT’s are being successfully applied toward neurodegenerative disorders, cancer, and even orphan diseases.
Everyone is familiar with how the COVID-19 pandemic promoted the development of messenger mRNA based vaccines. But we have seen significant, approved OT appearing since 2016. At that time Spinraza, an OT for spinal muscular atrophy, was released and was last year reported to have generated over $1.5Bn in sales. Then, in 2018 we saw the approval of Onpattro, a small-interfering RNA (siRNA) product to treat a polyneuropathy. And there are now over 25 approved OT therapies to-date [ 1 ].
As more products come online and as sales grow, we are seeing an increasing desire for greener manufacturing methods. ONs are bigger than small molecule drugs, but smaller than traditional biologics and advanced therapeutic medicinal products (ATMPs). Their manufacturing processes therefore exhibit an overlap between small molecules (e.g., using organic solvents) and biopharmaceuticals (e.g., in ISO8/7 environments). This gives the design for more sustainable manufacturing unique features. While there have been continual improvements, OT manufacturing still presents a relatively large environmental footprint. This is mainly due to its 1) use of large volumes of hazardous reagents and solvents, 2) relatively high process mass intensity (PMI), and 3) energy-intensive synthesis, purification, and isolation processes.
More Sustainable ON Production
Industry 4.0
Pharma 4.0 refers to the next wave of transformation in the pharmaceutical industry, incorporating advanced technologies such as artificial intelligence, data analytics, the Internet of Things (IoT), and automation to create a smart, connected, and data-driven manufacturing environment. It emphasizes the integration of digital technologies to enhance efficiency, quality, and innovation throughout the pharmaceutical production lifecycle. Pharma 4.0 is supporting process intensification, or the provision of more product using the same footprint, materials, and/or energy [ 2 ].
Cloud and digital twin-based resource management of materials, products, operations, personnel, and production schedules is providing such gains in green manufacturing as optimization of process efficiency, energy use timing, net energy demand, and product batches per facility or time [ 3 ]. Building information management (BIM) software are supporting 3D–7D facility modeling. Such modeling supports the optimization of many design parameters, including suite volume, process flow, classification level, energy and materials use, footprint, as well as heating, ventilation, and air conditioning (HVAC). And, the recent success of AI/ML in pharma operations, including in the empowerment of digital twins, promises even greater opportunities for both design and operational efficiency [ 4 ].
Facility design
ON manufacturing facilities can benefit from classical approaches to reducing energy demands generally employed in the industry, including reducing the extent of highly classified suites, optimizing energy demand, double commissioning, facility electrification, and employing green energy sources [ 5, 6, 7 ]. Because OT scale-up and production is such a dynamic and rapidly developing field, employment of current multimodal and flexible approaches in manufacturing suite and equipment design promises particular benefit [ 8 ].
Production processes
ONs are usually produced at-scale through solid-phase oligonucleotide synthesis (SPOS). As currently applied these employ large amounts of hazardous solvents and reagents and demonstrate a high carbon footprint. The need for greener reagents, solvents, and technologies was recognized by the FDA and the result was a request for better analytical methods and more stringent impurity specifications [ 9 ].
In a survey presented by AsahiKASEI at TIDES last August [ 10 ] a plurality of respondents placed a “High Priority” on sustainability initiatives in ON manufacturing. The essential principles of green manufacturing [ 11 ] are an excellent starting point for establishing compliant and environmentally responsible biomanufacturing.
Such generally applicable processes as inline conditioning (ILC) for buffer preparation is becoming a popular consideration that requires no bulk storage of buffer solutions, provides greatly configurable final formulations and volumes, and supports in-house preparation on demand.
But, because ON production employs process considerations common to both small-molecule and biopharmaceuticals, programs demand such special aspects as accommodation of different levels of biological, chemical, and solvent handling. Final efficiencies can only be accomplished following consideration of the specific operations in ON production methods.
Sponsors must consider both the specific needs of emerging OT therapeutics, as well as developments in production technologies promising a much smaller environmental footprint. Approaches here include alternative solvents, and such alternative production formats as improved solid-phase P(III) chemistries, tag-assisted liquid-phase, and continuous processes. Other promising approaches to consider include that some are experimenting with advanced stirred (or fluidized) bed methods yielding ONs of sufficient purity that further purification steps are not necessary.
And there is experimental evidence that we may be able to achieve sufficient efficiency using existing aqueous-based chemistries. Features here include compatibility with automated synthesis platforms and the potential for reducing organic solvent use. They typically involve phosphoramidite chemistry, but with modifications to make the reactions more water compatible. The primary advantage of aqueous over traditional solid-phase synthesis is elimination of organic solvents and dangerous chemicals [ 10 ].
Process intensification can increase the net mass of ON produced per time, materials, and footprint through e.g., either increased production efficiency or reduced loss in isolation and purification. From the point of view of PMI a 20-mer is reported to produce around 4300 kg of waste per single kg of API, not considering the burden from e.g., the preliminary production of reagents. However, such concepts emphasizing the atom economy of reactions are really not an efficient way to guide green production techniques, as they do not include such factors as the waste from other required steps.
Manufacturing mass intensity (MMI) can also be used in calculating, e.g., therapeutic dose-specific environmental burden from manufacturing. MMI considers mainly the amount of material used per unit of product manufactured. But while e.g., intensified OT manufacturing processes can reduce the MMI, such values should also not be taken as an absolute measure of environmental burden. Reasons include that in emphasizing raw material mass per se, production processes involving e.g., such organic solvents as acetonitrile and toluene have a far greater environmental impact compared to that of aqueous processes [ 12 ]. Based on the current MMI definition, a reduction in the use of water or aqueous buffers will be rated the same as a similar reduction in the use of organic solvents. The variety of solvents and other reagents used in ON processes complicates the overall environmental analysis, and the best performance index to apply is currently under development.
New production modes
Current solid-phase production requires thousands of liters of organic solvents, high MMIs (including dead volumes) as well as troublesome impurity and molecular variant generation. This results in such significant consequences as reduced production speed, increased cost, human toxicity, and environmental footprint.
Efforts are ongoing to reduce the environmental burden of ON manufacturing by changing the types of chemicals and solvents used, and through the elimination, reduction, or recycling of harmful materials. Early progress was made in replacing such hazardous reagents as dichloromethane (DCM) and tetrazole [ 13 ]. However, given the volume of such solvents as acetonitrile and toluene, as well as the nucleoside phosphoramidites and activators now used in ON processes, there is room for more improvement.
Work is ongoing to develop advanced scalable production methods based on aqueous buffers, perhaps using enzymes involved in natural synthesis of DNA, for an enzyme-catalyzed synthetic cycle. A related approach, enzyme-catalyzed templated ligation, uses DNA polymerases and reverse transcriptases to synthesize single-stranded ONs controlled by transient hybridization to neighboring strands.
Another interesting approach involves convergent synthesis of long-chain ONs from smaller “Blockmers”. That has been accomplished through traditional chemical means using organic solvents, but now can be done through an enzyme catalytic process in an aqueous buffer. The industry is also showing active interest in liquid-phase oligonucleotide synthesis (LPOS) and tag-assisted liquid-phase production. Along with hybrid solid/liquid and continuous production processes, the aim is to intensify processes and reduce harmful reagents.
Solvent recovery
Because manufacturing of OT is one of the few biopharma process requiring significant amounts of organic solvents (other than ethanol) in their manufacture, solvent recovery/recycling is especially important here. In the AshiKASEI survey mentioned above, when queried to rank their “Degree of Concern about Environmental Impact of ACN as a Waste Product of Oligonucleotide Manufacturing” a plurality responded, “Very Concerned”. And almost half of respondents (44%) report their organizations are likely to invest in ACN recycling technologies to reduce its environmental impact [ 10 ].
Solvent recovery and wastewater treatment of mixtures of organic and aqueous solvents involves such factors as the manufacturing scale, geographical setting, and safety concerns. A number of existing and emerging technologies including distillation, adsorption, and sieving offer options for dealing with contaminated water separation and polishing. However, two-phase separation offers the advantages of high capacity, efficiency, and relatively low energy demand compared to the alternatives.
Two-phase separation is based upon the principle that the solubility of a compound (in this case ACN) in water decreases in such conditions as reduced temperature or the presence of certain solutes. The decrease in the solubility of the organic compound leads to its precipitation or phase separation from the aqueous solution. Two-phase separation has traditionally been accomplished by cooling to subzero temperatures or salting out [ Figure 1 ]. In larger pharmaceutical production scale, these can suffer from such limitations as the amount of energy required, freezing of the samples, the concentrations of salt required, unwanted reactions, corrosion of equipment, and alteration of the environmental conditions (e.g., pH). There can also be such process considerations as mass transfer, fluid dynamics, fluid handling, and safety issues.
Two new technologies are contributing to the ability of establishing safe and practical two-phase separation at large scale.
1) Sugaring-out: a new phase separation method which overcomes some of the limitations in using salt. Additionally, glucose was found to be a better phase separating agent and retains almost all of some products in the aqueous phase [ 14 ].
2) Large-scale, continuous recovery of the phase-separated ACN organic phase using highly controlled continuous liquid-liquid/liquid-gas separators [ Figure 2 ]. Such instruments are based upon integrating selective wetting properties of a membrane with an in-line differential pressure controller.
In such instruments as Zaiput Flow Technologies’ SEP-40k, a biphasic stream (an aqueous phase and an organic phase) enters the separator, one phase will have an affinity for the membrane and fill the pores, and the other phase will be repelled and will not fill the pores [ 15 ]. Once the membrane pores are filled, a pressure differential is applied across the membrane. A key aspect of this technology is that it exploits differences in wettability and surface forces to accomplish the separation. As a result, the device can separate liquids with the same density, boiling point and emulsions in a continuous operation.
Toward a Greener Future
There are increasing demands to reduce the environmental burdens from ON manufacturing. A growing number of technologies and engineering advances in material, equipment, processing, and facility design should enable a greater sustainability in future OT manufacturing.
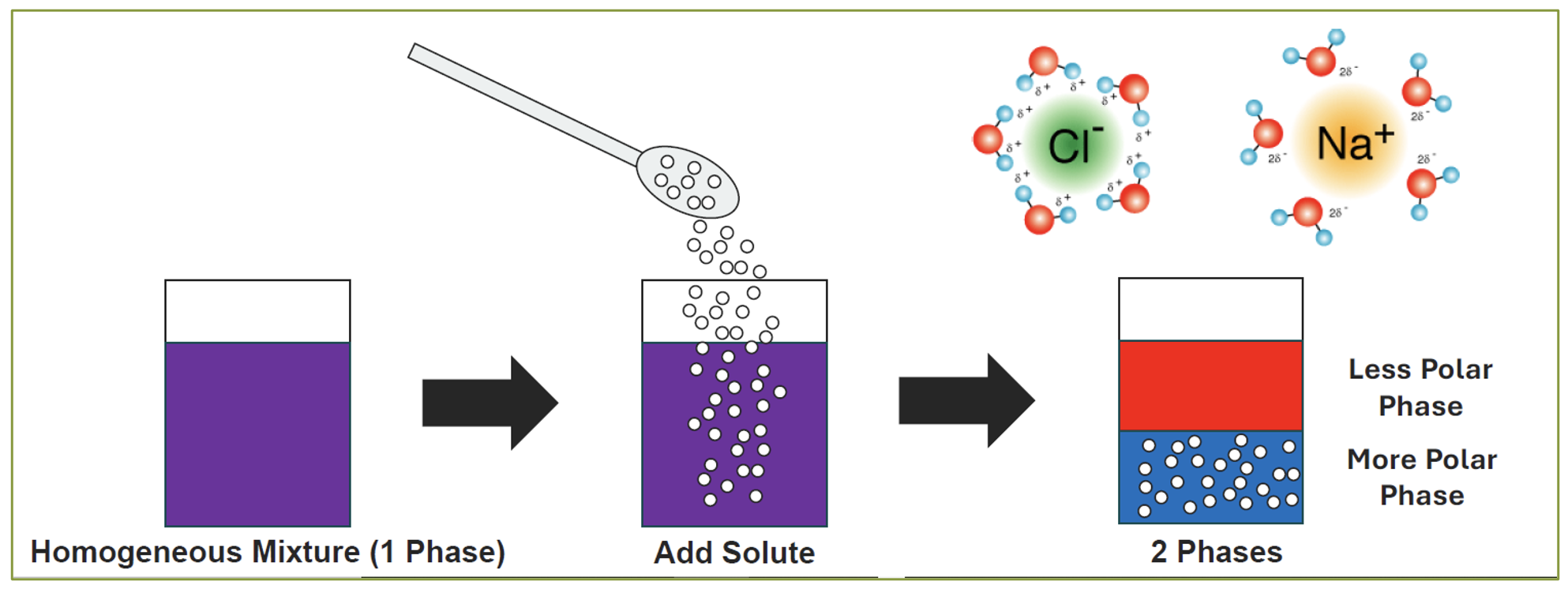
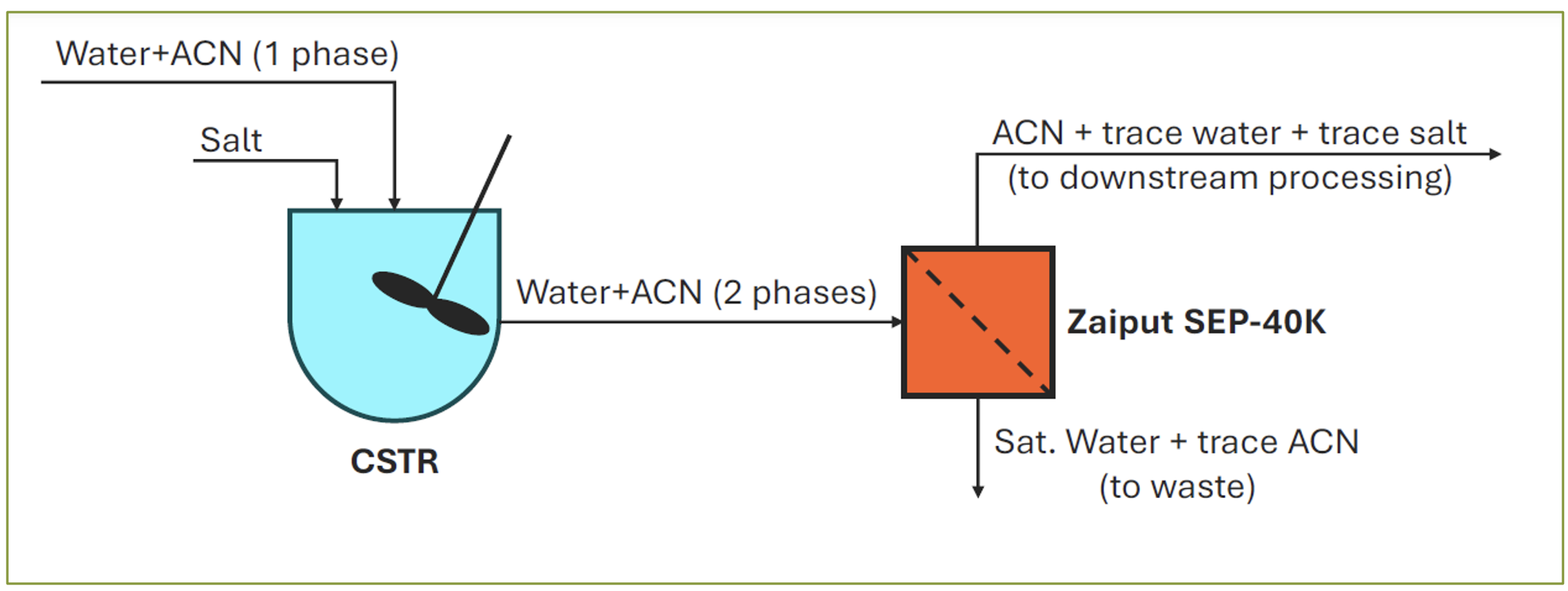
About the Author
Bill is currently the Strategic Solutions Leader for Arcadis, having over 20 years experience leading teams in biotechnology product and process development. He now publishes oral papers, print articles, and book chapters on such topics as ATMP, AI/ML in pharma, and sustainable biomanufacturing practices. Recently his work has been acknowledged in the 2022 APEX Award for Publication Excellence in the Technical & Technology Writing category and the 2023 ISPE Roger F. Sherwood Article of the Year award. He currently enjoys serving on such committees as the BioProcess International Editorial Advisory Board, and the AFDO/RAPS Healthcare Products Collaborative Artificial Intelligence in Operations Team. He currently has an h-index of 17 and an i10 index of 29.
Footnotes
-
1. https://www.iqvia.com/blogs/2023/11/rna-therapeutics-rewriting-the-script-of-medical-treatments
-
2. Boodhoo VK, et al. Bioprocess Intensification: A Route to Efficient and Sustainable Biocatalytic Transformations for the Future. Chem. Eng. Proc. Proc. Intens. 172, 2022: 108793; https://doi.org/10.1016/j.cep.2022.108793
-
3. Whitford WG. Chapter 4: Process Control, Intensification, and Digitalisation in Continuous Biomanufacturing. Bioprocess Intensification: Technologies and Goals. Subramanian G, Ed. Wiley VCH: Germany, 24 December 2021; https://doi.org/10.1002/9783527827343.ch4.
-
4. Manzano, T., and Whitford, W. (2023). “AI applications for multivariate control in drug manufacturing, in A Handbook of Artificial Intelligence,” in Drug Delivery, ed. A. Philip, A. Shahiwala, M. Rashid, M. Faiyazuddin (Academic Press), 55-82.
-
5. Whitford, W, Heffernan, E, Kelly A. Environmental Sustainability in Biopharmaceutical Facility Design. Pharm. Eng. March–April 2023; https://ispe.org/pharmaceutical-engineering/march-april-2023/environmental-sustainability-biopharmaceutical-facility.
-
6. Whitford, W, Jones, D, Kinnane, S. Biomanufacturing Design: Reducing the Environmental Burden Curr. Opin. Biotechnol. 76, 2022:102717; https://doi.org/10.1016/j.copbio.2022.102717.
-
7. Whitford, W, & Murtagh, Flexible Facility Design for Sustainable Oligo Manufacturing, in eBook: Oligos and mRNA — Manufacturing in Facilities Around the World, BioProcess International, Wednesday, July 26, 2023
-
8. Judd, S and Whitford, W, Supporting Cell & Gene Therapy through Multimodal & Flexible Facilities, Pharm. Eng., November / December 2022, https://ispe.org/pharmaceutical-engineering/november-december-2022/
-
9. From green innovations in oligopeptide to oligonucleotide sustainable synthesis: differences and synergies in TIDES chemistry. https://pubs.rsc.org/en/content/articlehtml/2023/gc/d2gc04547h DOI: 10.1039/D2GC04547H
-
10. Sustainability Practices in Oligonucleotide Manufacturing ONLINE SURVEY https://www.bioanalysis-zone.com/events/tides-usa-oligonucleotide-and-peptide-therapeutics-2023/
-
11. Anastas, P., & Zimmerman, J. (2003). Design through the Twelve Principles of Green Engineering. Environmental Science and Technology, 37(5), 94A-101A.
-
12. Benison CH, Payne PR. Manufacturing Mass Intensity: 15 Years of Process Mass Intensity and Development of the Metric into Plant Cleaning and Beyond. Curr. Res. Green Sust. Chem. 5, 2022: 100229; https://www.sciencedirect.com/science/article/pii/S2666086521001764
-
13. Sanghvi YS, et al. Applications of Green Chemistry in the Manufacture of Oligonucleotide Drugs. Pure Appl. Chem. 73(1) 2001: 175–180; https://doi.org/10.1351/pac200173010175
-
14. Pradip B. Dhamole, Prafulla Mahajan, Hao Feng, Sugaring out: A new method for removal of acetonitrile from preparative RP-HPLC eluent for protein purification, Process Biochemistry Volume 45, Issue 10, October 2010, Pages 1672-1676. Sugaring out: A new method for removal of acetonitrile from preparative RP-HPLC eluent for protein purification - ScienceDirect
-
15. Zaiput Flow Technologies, https://www.zaiput.com/product/z-fox-batch-extraction-platform/