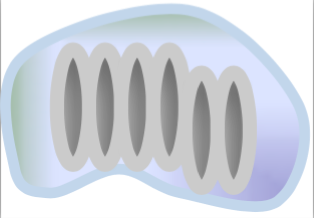
All About Organoids
Held this year in Boston MA, the International Society for Stem Cell Research (ISSCR) Conference 2017 brought together more than 4,000 researchers, bioethicists, clinicians, and industry professionals from all over the globe to discuss the latest discoveries and technologies in Stem Cell Research and their contributions to advancing regenerative medicine. This year, the plenary session topics included an exciting and rapidly expanding area of research—organoids. An organoid, in the most basic sense, is a miniaturized and simplified version of an organ produced in vitro in three dimensions that show realistic micro-anatomy. Unlike two dimensional (2D) cell cultures, organoids enable three-dimensional (3D) cell growth, movement and differentiation, making this technology an effective model for understanding organ development, tissue morphogenesis and the genetic or molecular basis of diseases (Xinaris et al, 2015).
Speakers at ISSCR presented basic and applied research on organoids and discussed how these structures are providing insights into disease, tissue repair, and applications for precision medicine.
What is an Organoid?
Fatehullah et al (2016) define an organoid as an in vitro 3D cellular cluster derived from primary tissue (lineage-restricted adult stem cells), embryonic stem cells (ESCs), or induced pluripotent stem cells (iPSCs), capable of self-renewal and self-organization, and exhibiting similar organ functionality as the tissue of origin.
Many publications over the years have demonstrated that culturing cells in a 2D flat layer in a dish is not representative of the 3D organization of cells and extracellular matrix (ECM) within tissues and organs. Cells engage in complex cell-cell and cell-matrix interactions and this ‘cross-talk’ is crucial for mimicking in vivo environments (Akkerman, 2017). Without these connections, cells can lose their distinctive phenotype and react atypically to external cues, creating challenges when 2D-cultured cells are used for drug screening applications. This highlights the importance of culturing cells in 3D for recapitulating the in vivo state to study signaling, differentiation, and to serve as a model for drug screening and development.
Organoid technology has rapidly improved since the early 2010s, dubbed one of the biggest scientific advancements of 2013 by The Scientist. Researchers have devised different methods for 3D culturing of stem cells to generate organoids resembling a number of organs in animals and humans including gut, brain, liver, thymus, thyroid, lung, pancreas, heart and more.
Although still small in size (≤ 3mm in diameter), organoids are relatively stable model systems of animal and human organs and tissues that are amenable to long-term cultivation and manipulation (Akkerman, 2017). In conjunction with advances in reprogramming technology and gene editing methods, organoids allow unprecedented insight into human development, act as disease models, be utilized for drug-screening platforms, and may one day be used to generate tissue for transplantation.
Organoid Applications
Developmental Biology
For developmental biologists, organoids offer unique insight into how cells self-organize to become organs. Intestinal organoids generated from adult stem cells of the intestinal epithelium are among the first reported (Sato et al., 2009). In 2009, at the Hubrecht Institute in Utrecht, Netherlands, Dr. Hans Clevers’ group demonstrated the formation of 3D intestinal organoids from mouse Lgr5 (Leucine-rich repeat-containing G-protein coupled receptor 5)-expressing intestinal stem cells isolated from the base of the crypt. When cultured in a Matrigel™ matrix with growth factors EGF (epidermal growth factor), R-spondin-1(Wnt agonist) and Noggin (Bone Morphogenetic Protein inhibitor), they were able to recapitulate the crypt and villi of the mouse small intestine with high fidelity (Figure 1).
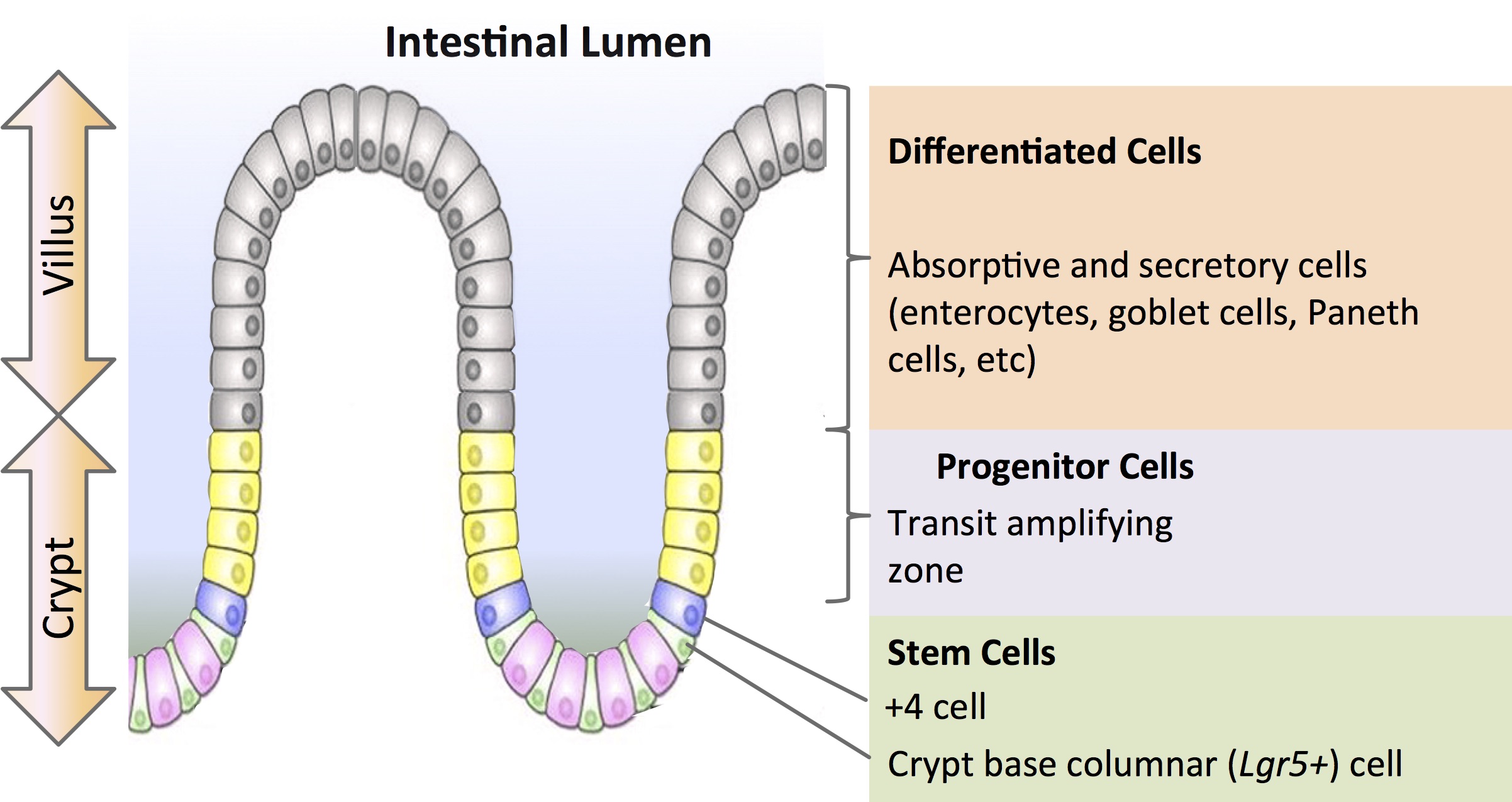
During his talk at ISSCR, Dr. Clevers outlined the work done by Paul Tetteh (2016) where organoids were used to study stem cell replacement in the mouse intestinal niche. A stem cell niche can be defined as “a specific location in a tissue where stem cells can reside for an indefinite period of time and produce progeny cells while self-renewing” (Umar, 2010). It has been demonstrated that the intestinal crypts display robust regeneration upon injury. In particular, the secretory precursors that give rise to Paneth and enteroendocrine cells can revert to a multipotent state upon loss of resident Lgr5+ stem cells in the niche (Buczacki, 2013). Tetteh et al sought to establish if the abundant and highly proliferative enterocyte progenitors—residing above the stem cell niche— displayed similar plasticity. Using labeling experiments, they successfully showed that these Alpi+ (Alkaline phosphate intestinal gene) progenitors could de-differentiate to become Lgr5+ intestinal stem cells when the ones in the niche are ablated by down-regulating enterocyte-specific genes and upregulating Lgr5 stem-cell-specific genes. The enterocyte progenitors effectively regain their ‘stemness’ to repopulate the stem cell niche.
Researchers continue to be amazed by the impact of this powerful technology in furthering our knowledge of regenerative populations present in many tissues and the mechanisms to maintain homeostasis (Little, 2016). Organoid technology is also expanding our understanding of human disease and potential therapeutics as researchers develop novel methods to use these 3D systems.
Disease Modeling
Human organoids reflect key structural and functional properties of organs such as kidney, lung, gut, brain and retina making them valuable for human disease modeling. In particular for brain disorders where there is an unmet need for an in vitro human model. The unique complexity of human brain development has proved a challenge to study with animal models. Dr. Jüergen Knoblich delved into this topic during his talk at ISSCR entitled ‘Cerebral Organoids: Modeling human brain development and tumorigenesis in stem cell-derived 3D culture’. Specifically, he described the pioneering work by Lancaster (2013) to form cerebral organoids in suspension culture (Figure 2).
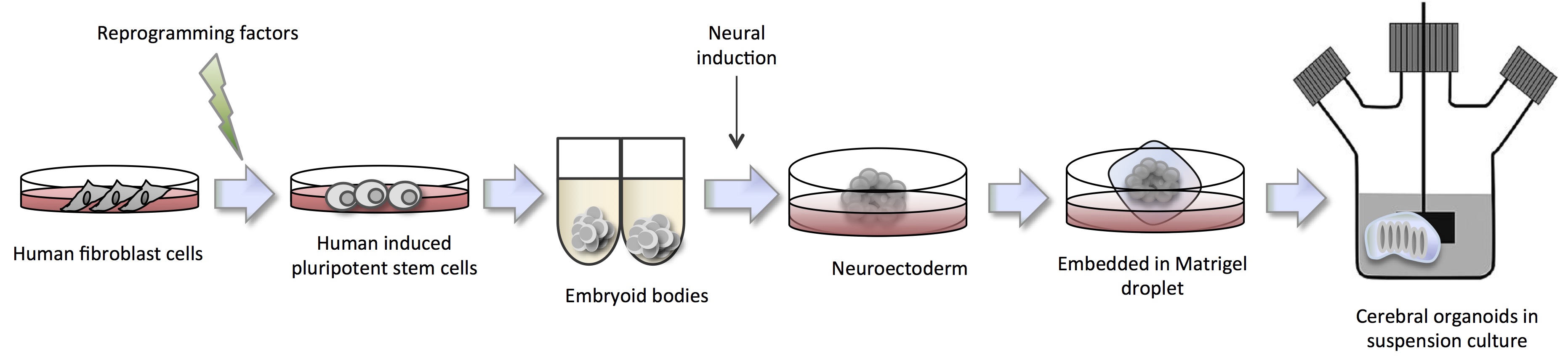
The cerebral organoids were used to model human microcephaly, a condition that is poorly recapitulated in mouse model systems. Microcephaly is a disorder where the brain does not develop properly resulting in a significantly smaller than normal head size causing intellectual disabilities, poor motor function and more. In collaboration with Andrew Jackson of the University of Edinburgh, Lancaster identified a patient showing severe microcephaly found to have a truncating mutation in the CDK5RAP2 (CDK5 regulatory subunit-associated protein 2) gene, which is necessary for progenitor cell maintenance during neural development. The corresponding iPSCs formed significant smaller cerebral organoids with reduced neuro-epithelial tissues, fewer progenitor cell regions and large domains of differentiated and mature neurons, suggesting that premature differentiation causes the in vivo establishment of the diseased phenotype. Involvement of the CDK5RAP2 gene was further cemented when researchers re-introduced the functional protein and were able to restore organoid development to normal.
Cerebral organoids from pluripotent stem cells are especially useful because their growth emulates characteristics of human brain development. If something goes wrong along the way developmentally, it is observable as the organoids form and mature, allowing researchers to investigate potential causes, method-of-action, and possibly identify drug targets for treatment (Juskalian, 2015).
Drug Screening/Drug Therapy
Because human organoids can mimic the physiological responses of their organ counterparts, they are more relevant for predicting drug toxicity than animal models. In one such example, Dr. Melissa Little and colleagues at the Murdoch Childrens Research Institute in Melbourne, Australia established a protocol to generate kidney organoids from hiPSCs containing all the functional components of the kidney (Takasato et al., 2015). When these organoids were challenged with cisplatin, a nephrotoxic drug, they demonstrated proximal renal tube damage at drug dosages comparable to that observed in a clinical setting making them useful models for screening pharmaceuticals. Additionally, organoid models could be used for high throughput drug screens which would not be feasible with animal-based toxicity testing.
The intestinal organoid model is already proving its value in clinic for cystic fibrosis (CF). CF is an autosomal recessive disease caused by mutations in the cystic fibrosis transmembrane conductance regulator (CFTR) gene which makes it difficult for chloride channels to open in the epithelial cells of many organs leading to a buildup of thick, sticky mucus in the lungs and guts causing airway obstruction and infection.
KALYDECO® is a treatment for CF made by Vertex Pharmaceuticals Inc., approved in 2012, which is a small molecule CFTR modulator. It functions by opening up the restricted ion channels allowing easier movement of salt and water. However, only those patients with the GD551D CFTR mutation (estimated at ~4% of all CF patients) respond effectively to the drug. This combined with the high cost of KALYDECO® necessitated a model to screen patient’s response to drug quickly and cheaply before it is prescribed for treatment.
Intestinal organoids generated from rectal tissue samples of CF patients were used to test their responsiveness to KALYDECO®. This work was done by the Clevers group (Dekkers, 2013) in collaboration with Jeffrey Beekman and Kors van der Ent at Wilhelmina Children’s Hospital in Utrecht, Netherlands. The CTFR can be activated forskolin, a pharmacological agent raises intracellular cyclic AMP levels, causing organoids from healthy patients to swell as fluid moves into the lumen (Cain, 2013); an effect not observed in CF patient organoids. If a patient is responsive to KALYDECO®, their organoids would swell— a sign that CTFR function was restored and the cells are moving salt and water effectively. This intestinal organoid model is a rapid and quantitative assay for CFTR function that is being used to test and screen patients for other prospective CF therapies.
Organoid Limitations
Today’s organoids, while still rudimentary, have already facilitated scientific discoveries unattainable with animal models or through study of primary tissues. However, there are still some major limitations to be addressed.
At ISSCR, Dr. Jüergen Knoblich described work by Madeline Lancaster (2016) where bioengineered scaffolds are helping to form brain organoids in a more reproducible fashion compared to earlier versions which relied on the cells to self-organize in 3D. The scaffolds are made with polylactic-co-glycolic acid (PLGA) microfibers helps direct growth so the organoids can form cerebral cortical tissue more reproducible (dubbed enCORs-engineered cerebral organoids) while still allowing for normal differentiation of the tissue and opportunity for neural migration.
Another challenge with organoid technology is optimizing growth conditions. The size of the organoids is currently constrained by the maximum distance of diffusion of nutrients and gas exchange. Larger sized organoids with increased complexity organoids may be possible in the future as the methods for nutrient supply improve (i.e. spinning bioreactors to facilitate higher extents of diffusion; Lancaster, 2013). Additionally, researchers are investigating co-culturing organoids with endothelial cells to develop vascularization (Yin, 2016).
Although organoid cultures can be grown and maintained for several months, their ability to mature in vitro is unclear which impacts their utility for developmental research, as organoids may only recapitulate the first few months of development, but not the stages beyond (Akkerman, 2017); therefore potentially lacking in cell types of interest for researchers.
Perhaps the biggest shortcoming with organoid technology is that they fail to replicate some of the most important features of real organs. An organ has support scaffolds, blood vessels to deliver nutrients and signal molecules, and interacts with and responds to cues from other organs in the body. A DARPA (Defense Advanced Research Projects Agency)-funded “body-on-a-chip” could solve the problem by connecting organoids with a blood substitute, but the approach is still experimental (Lewis, 2016).
Future Prospects and Final Remarks
Organoid technology has the potential to change the way we do biomedical research, impacting many subjects that have been of intense ethical debate—from animal experimentation to personalized medicine, transplantation, and Gene Therapy (Bredenoord, 2017). For instance, the ‘mini brain’ models, developed by Thomas Hartung’s group (Johns Hopkins University, Baltimore MD) can spontaneous generate electrical activity, producing brain waves. This is a long way off from having a functional “brain in a dish” because these mini brains lack certain cell types and connections to other organs but as the technology advances and organoids become more complex, where is the ethical threshold? Growing organoids of human sex glands or reproductive organs in a lab dish will fuel concerns, notes Stanford University bioethical law professor Henry Greely. The repercussions of such research will need to be weighed by governing and regulatory bodies and balanced with the demand from patients for novel therapies (particularly for transplantation and gene therapies).
While organoid field is still relatively new, organoids have emerged as a powerful tool to complement existing animal and cell line models offering up unprecedented insight into human development and disease. This opens up innovative approaches to medical research, drug discovery, toxicology testing and for advancing precision and regenerative medicine bearing great promise for the future.
Other ISSCR 2017 Related Articles:
Efficient Large-Scale Expansion of MSCs for Translational Medicine and Research Use
Footnotes
-
1. Akkerman, N. and Defize, L. H.K. Dawn of the organoid era: 3D tissue and organ cultures revolutionize the study of development, disease, and regeneration (2017). BioEssays 39(4): n/a. doi:10.1002/bies.201600244
-
2. Bredenoord, A et al. Human tissues in a dish: The research and ethical implications of organoid technology (2017). Science 355 (6322) doi: 10.1126/science.aaf9414
-
3. Buczacki, SJ et al. Intestinal label-retaining cells are secretory precursors expressing Lgr5 (2013). Nature 495(7439):65-9. doi: 10.1038/nature11965
-
4. Cain, C. Personalizing cystic fibrosis in vitro (2013, June 13) SciBX 6(23); doi:10.1038/scibx.2013.564
-
5. Clevers, H. Modeling Development and Disease with Organoids (2016). Cell 165(7):1586-97. doi: http://dx.doi.org/10.1016/j.cell.2016.05.082
-
6. Dekkers, J.F. et al. A functional CFTR assay using primary cystic fibrosis intestinal organoids (2013) Nat. Med. 19: 939–45 doi:10.1038/nm.3201
-
7. Fatehullah, A et al. Organoids as an in vitro model of human development and disease (2016). Nature Cell Biology 18(3): 246–254. doi:10.1038/ncb3312
-
8. Gent, E. The Ethics of Organoids: Scientists Weigh in on New Mini-Organs (2017, Jan 27). SingularityHub Retrieved from URL: https://singularityhub.com/2017/01/27/the-ethics-of-organoids-scientists-weigh-in-on-new-mini-organs/ Accessed August 20, 2017.
-
9. Juskalian, R. Brain Organoids: A new method for growing human brain cells could unlock the mysteries of dementia, mental illness, and other neurological disorders (2015). MIT Technology Review. Retrieved from URL: https://www.technologyreview.com/s/535006/brain-organoids/ Accessed August 20, 2017.
-
10. Lancaster M, et al. Cerebral organoids model human brain development and microcephaly (2013). Nature 501(7467):373-9. doi: 10.1038/nature12517
-
11. Lancaster, M et al. Guided self-organization recapitulates tissue architecture in a bioengineered brain organoid model (2016) bioRxiv doi: 10.1101/049346
-
12. Lewis, T. Will Organs-in-a-Dish Ever Replace Animal Models? (2016, July 19) The Scientist. Retrieved from URL: http://www.the-scientist.com/?articles.view/articleNo/46588/title/Will-Organs-in-a-Dish-Ever-Replace-Animal-Models-/ Accessed August 20, 2017.
-
13. Little, MH. Closing the circle: from organoids back to development (2016). Development 143: 905-906; doi: 10.1242/dev.136150
-
14. Qian X et al. Brain-Region-Specific Organoids Using Mini-bioreactors for Modeling ZIKV Exposure (2016). Cell. 165(5):1238-54. doi: 10.1016/j.cell.2016.04.032
-
15. Sato,T et al. Single Lgr5 stem cells build crypt-villus structures in vitro without a mesenchymal niche (2009). Nature 459, 262-265. doi:10.1038/nature07935
-
16. Servick, K. Lab-grown mini-organs help model disease, test new drugs (2017, March 14). The Scientist. Retrieved from URL: http://www.sciencemag.org/news/2017/03/lab-grown-mini-organs-help-model-disease-test-new-drugs Accessed August 20, 2017.
-
17. Takasato,M et al. Kidney organoids from human iPS cells contain multiple lineages and model human nephrogenesis (2015) .Nature 526(7574):564-8. doi: 10.1038/nature15695
-
18. Tetteh, P et al. Replacement of Lost Lgr5-Positive Stem Cells through Plasticity of Their Enterocyte-Lineage Daughters (2016). Cell Stem Cell 18(2): 203–13. doi: http://dx.doi.org/10.1016/j.stem.2016.01.001
-
19. Umar, S. Intestinal Stem Cells (2010). Curr Gastroenterol Rep.12(5): 340–348. doi: 10.1007/s11894-010-0130-3
-
20. Willyard, C. Rise of the Organoids (2015, July 30). Nature News 523 (7562): 520-22. doi:10.1038/523520a
-
21. Xinaris, C et al. Organoid Models and Applications in Biomedical Research (2015). Nephron 130(3):191–199 doi: 10.1159/000433566
-
22. Yin, X et al. Stem Cell Organoid Engineering (2016). Cell Stem Cell 18(1): 25–38. doi: 10.1016/j.stem.2015.12.005