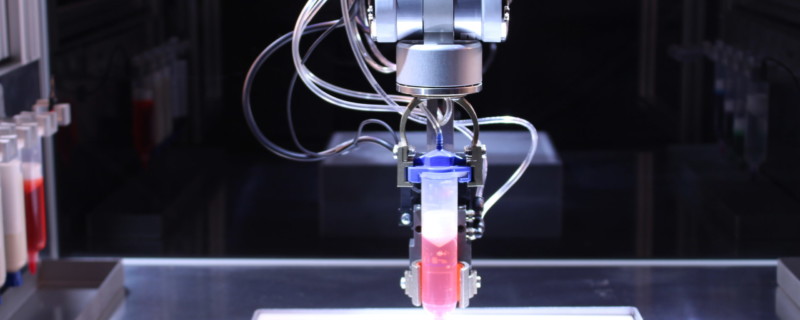
Another Perspective on 4D Bioprinting
A Guest Blog by William Whitford, Strategic Solutions Leader, BioProcess, GE Healthcare Life Sciences
Introduction
3D bioprinting has been promoted and even implemented to varying degrees for more than a decade [1]. Proposed applications abound, although for some their component technologies remain to be completely established [2]. The recent invention of 4D printing naturally points to the general concept of 4D bioprinting. However, the principles and terms inherent to the concept of 4D bioprinting are still in early development. Here an outline of the proposed implementations of 4D bioprinting is reviewed and a comprehensive definition introduced.
3D Printing
3D printing refers to various processes used to synthesize a three-dimensional object [3]. It is also known as additive manufacturing (AM). A key concept here is that layers of material are sequentially deposited under computer control to create a solid object. [4] The technology is already successful with many applications in full operation and employing many diverse materials.
4D Printing
4D printing is a new process for printing of “smart” products. These are printed objects that, for example, respond to environmental conditions by such conformational changes as deforming, oscillating, shrinking, twisting or growing. The environmental variations initiating these changes include temperature, moisture, electrical pulse, light (or other direct energy input), air pressure/shear/turbulence, stress, etc. When a 4D printed drain cover is exposed to water, for example, it could warp and open-up or un-seal the drain. Here the bilayer printed material drain cover is flat when dry, but when it becomes wet warps or curves, generating a poor seal around the drain that allows water to flow out and escape. For the most part, reversibility and cyclability of the activity is understood. However, one can clearly envision a 4D printed object that is specifically engineered to deform to a particular shape through a defined environmental stimulus only once. Or one that is engineered with a capability to cycle multiple times, but with that capability intended to be employed only once. This adaptability and dynamic response can be very simple, such as in the drain example, or much more complex, such as in complex adaptive products like responsive garments or even material-integral robotic-like behavior.
But definitions can be tricky. For example, there are many other smart objects that respond by design to environmental input, such as photochromic eyeglass lenses. But, these are not printed. 3D printed thermoplastic objects may bend or deform under pressure or heat, but this is not a designed or functional change. Hence, a more specific definition of these particular smart objects might be “single multi-material printed objects that demonstrate engineered actuation, sensing or material logic resulting in its physical transformation in response to designed user-demands or changing environment”.
The actual 4D printing process begins with the 3D printing of an object composed of multiple, “responsive” materials. These materials can be composed of almost anything─ for example, metals, plastics or glasses that determine the shape transformation, by material state-change, that the printed object will make. This builds “solid-state” functionality and change mechanisms directly into the printed objects.
4D Bioprinting
4D bioprinting is analogous to 4D printing in that it is the printing of smart, environmentally responsive biological structures, tissues and organs. 4D bioprinting begins with the printing of multiple cells or biological matrices resulting in structures that
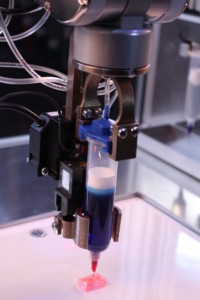
undergo subsequent, designed and anticipated (not spontaneous) but self-originated development in response to an environment. Four distinct types of 4D bioprinting have been proposed.
One type, named “shape change”, denotes a 4D printed substrate, composed of smart biopolymer (and cells?) which changes its 3D configuration (or shape) upon stimulation. A second type, called “size change”, is defined as a type of in vivo bioprinting where a 3D printed device (eg, of a hydrogel composition) is implanted in the body, and subsequent biological activities leave tissue where the (now absorbed) implant once existed. In a third approach, termed “pattern change”, micro-droplets of cells are printed in an assembly of a particular pattern or orientation, and that pattern is subsequently stimulated to a pre-envisioned new pattern. Similar to 4D printing, the preceding three types of bioprinting all involve an engineered and predicted change in the assemblies post-printing physical shape, size or pattern [5].
A fourth type of bioprinting has also been suggested [6]. This type’s inspiration is that, unlike in 4D printing, 4D bioprinting contains the unique element of a dynamic, biological development and response. In 4D printing a valve can twist and open, an airfoil can bend and adapt, and a window shade can deform and open. However, in the 4D bioprinting environment, significant, functional and pre-engineered changes can be designed to occur which are dependent upon and/or result in non-structural but highly biologically relevant activity. Such non-structural or physical-organizational yet highly understood and predictable changes can be designed into 4D bioprinted structures. It should be understood that these cellular and biological developments can have direct consequences on the printed product’s aggregate biological phenotype, function or even shape and structure.
Examples include component cells responding to such environmental actuators/mediators as
- immunological signals and input
- adjacent cell or basement membranes
- juxtacrines / paracrines / endocrines, etc.
- physicochemical “stress” or response inducers
- differentiation signals and epigenetic modulators
- nutritional / factor components and their gradients
Such non-structural 4D bioprinting offers post printing
- cellular “CD” development and display
- cell receptor characteristic development
- cellular type differentiation / adaptation
- functionality development in organoid culture
- cellular polarization influencing self-organization
- dynamic, pluri-consequential or reversible responses
- mutations and chromosome silencing in transplantation chimera
Examples of anticipated, but purely biological responses include
- multipotent in situ cell differentiation
- restricted chimerism with human naïve iPSCs
- in situ tissue vascularization or organ development
- admixtures determining the potency of cells in organ development
It has been proposed that 4D bioprinting is currently constituted by only the first three, more physical, developments and not the fourth, more biological-change oriented developments outlined above. However, it can seem incomplete to allow only changes in gross structure and physical organization in the definition of a construct or assembly whose hallmark motivation of change is derived from biology and changes at the cellular level. And, the recent advances in therapeutic tissue/organoid/organ chimera would seem to demand at least a clear decision as to if merely shape/orientation/patterning is to be included in the definition of 4D bioprinting [7].
As many of the changes involved in the fourth type occur initially at the cellular level, it could be argued that they are not descriptive of a printed product or “assembly” of cells. However, many of the particular cellular developments above concurrently establish a “construct” or “tissue” characteristic, often referred to by a similar designator. For example, the in situ development of a heightened differentiation of cellular components in a particular tissue might result in significant cellular polarization and morphologic changes en masse. Description of merely that tissue “organizational” state and macro-physical change seems possibly an over simplification and potentially an omission of the true and fully understood nature of the events occurring.
A simple scenario will illustrate the import of this last, and more cell biology, type of 4D printing. One can envision a future where a number of highly understood bioinks are prepared with a hydrogel matrix dispersed in, for example, HyCloneTM AdvanceSTEM DMEM4SC. Each bioink would variously contain differentiated autologous fibroblasts and keratinocytes as well as precursors of endothelial cells, melanocytes, Schwann cells and hair follicle cells. These inks are thereafter employed in 4D printing of sheets of structured cell constructs. Once implanted, these constructs would be anticipated to generally undergo a predicted in situ process involving structural and organizational changes.
However, such constructs might also be specifically designed to experience number of predicted cellular differentiation, adaptive, polarization and signaling pathway induced responses. Then, these anticipated biochemical responses would support number of changes─ including (but not limited to) those directly providing such tissue-like characteristics as intercellular adhesion, communication and vascularization.
4D Bioprinting Definition
Considering all of the above, we propose the following definition for 4D bioprinting:
The construction of individual multi-material printed objects from either living or preliminary substrates for medical or biotechnological applications when they are specifically engineered to respond in anticipated ways to user-demands or a changing environment by self-actuated morphogenesis, cellular differentiation, tissue patterning, defined biological characteristic alteration or functionality development.
Conclusion
4D bioprinting is analogous to 4D printing in that it is the printing of “smart” biological structures. It begins with the printing of cells or matrices that result in structures that undergo designed and self-actuated biological responses. By any definition, some examples of such a technology have already been accomplished. These examples range from current therapeutic and diagnostic tissues to experimental vascularized or chimeric constructs. However, as the practice of even 3D bioprinting is still in its infancy, the composition and functional boundaries of true 4D bioprinting remains understandably in debate.
References
- Sargent B, 3D Printing and 3D Bioprinting – Changing the Healthcare Landscape, July 2, 2015
- Golson K, 3D Bioprinting – Research Successes, Challenges, and Future Possibilities, The Cell Culture Dish, July 9, 2015.
- Excell J. “The rise of additive manufacturing”. The Engineer. Retrieved 2013-10-30.
- Jump up “3D Printer Technology – Animation of layering”. Create It Real. Retrieved 2012-01-31.
- An J, Chua K and Mironov V, 2016, A Perspective on 4D Bioprinting. International Journal of Bioprinting, vol.2(1): x–x. http://dx.doi.org/10.18063/IJB.2016.01.003.
- Whitford W, Development Strategies for Bioprinting Media and Buffers, International Bioprinting Congress, 9-10 July 2015, Singapore.
- Regalado A, Human-Animal Chimeras Are Gestating on U.S. Research Farms, Biomedical News, MIT Technology Review, January 6, 2016.
Author Information
William G. Whitford
Strategic Solutions Leader, BioProcess
GE Healthcare Life Sciences
925 West 1800 South Logan, Utah 84321
Email:bill.whitford at ge.com