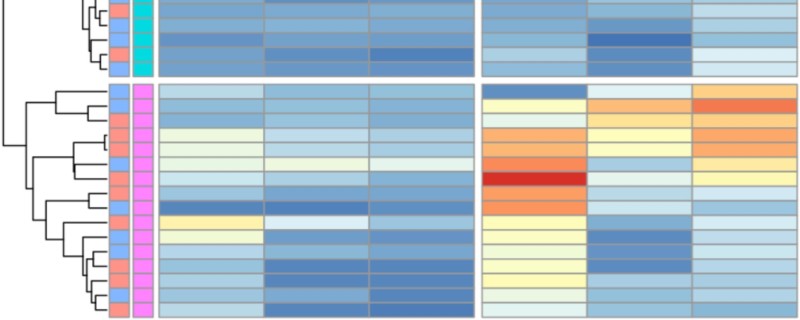
Bioprocess Intensification – A discussion on the what, the how, and the why
Bioprocess intensification is a term that we hear often, but it is difficult to define or rather there are often several definitions depending on the perspective of the author. That said, it is generally considered a toolbox of strategies to improve the biomanufacturing process and make it more efficient. What is meant by “efficient” is a metric that has to be defined by the group working to intensify the process. It could be increasing productivity, lowering manufacturing costs, better utilization of facility space, reducing timelines, improving product quality or all of the above and more.
I was recently able to interview William Whitford, an expert in the field of upstream bioprocessing, about what bioprocess intensification means to biomanufacturing, the tools and technologies we are using to apply it, and what is driving its implementation. A transcript of our interview follows.
What does the term Bioprocess Intensification mean?
This is a difficult question for four reasons. First, the number of different types of new biological products, from things such as exosomes to DNA vaccines, makes any generalization regarding new biomanufacturing initiatives difficult. Secondly, bioprocess intensification efforts include many different technologies, affecting these many products as they support diverse analytics, diagnostics and therapeutic applications. Thirdly, there are so many advances in biomanufacturing, that range from process development techniques to new manufacturing platforms, equipment, and facilities. And, developments in related science, technology, and systems are equally remarkable.
The fourth concern regards just what improvements in a process should be addressed by the title “bioprocess intensification.” Some have applied it to process improvements that result in higher product quality or regulatory ease. But many use it as only when referring to getting more product out of a process, or put another way, by producing more product per cell, time, volume, footprint or cost. When used this way, bioprocess intensification refers to any activity that increases such common metrics as the cell-specific, volumetric or facility productivity (among others). This could be accomplished by, for example, producing more product per time period upstream or retaining more quality product downstream. Defined this way, bioprocess intensification a subordinate or supporting technology to the so-called Next-Gen or Factory-of-the-Future initiatives, and a sister or corollary technology to such initiatives as Continuous or Digital Biomanufacturing.
A final point here is that many process improvements often affect values and metrics which were not the main intent, and so when critically examined, their total effects overlap quite a bit. For example, a process improvement intended to improve product quality can, upon review, reduce failed batches and thereby increase a plant’s annual productivity. It can also support greater regulatory audit ease. But we try to limit the stated scope of improvement activities to their primary and intended goals.
So, to wrap up, bioprocess intensification is increasing productivity by any metric.
It is quite complex and there is more to the definition than first appears. This inspires the question – Why is bioprocess intensification important today?
I see this as being driven by two emerging trends. The first is that years ago, cost control and process efficiency concerns were not top priorities for biopharmaceutical manufacturers, but that has been changing of late. Such evolving industry trends as biosimilar licensing, in-country for-country production, and the capabilities afforded by single use production systems and modular prefabricated facilities have all contributed to a need for process economy and efficiency.
Relatedly, many different industry sectors now active in “bioproduction” require large quantities of product, due to e.g., dosage mass, sourcing constraints or global demand. And, there is the maturing of the industry. Often there is a pressure to quickly develop a functional first-generation process, and only later does attention turn to such goals as process capacity, robustness, consistency, efficiency, manufacturing flexibility and sustainability.
But, as bioprocessing presents such challenges as high labor and material costs and a demand for extensive experimental efforts, the opportunity and potential for intensification is great. Finally, for such reasons as regulatory and quality constraints, biomanufacturing has been slow to incorporate efficiencies from such newer manufacturing initiatives as digitalization (“Industry 4.0”), automation, standardization, miniaturization and operations integration.
What tools and technologies are being used to accomplish all of this?
Biomanufacturing has evolved into a remarkably dynamic state. Considering the new product types and applications, new manufacturing processes and support technologies, and the new facility designs and fabrication methods, it’s difficult to even catalog the developments arising.
We might start with Asset Performance Management (APM), which in general can provide better control over production, materials management, maintenance and repairs. Data analysis can improve the performance of existing facilities while minimizing costs and risk.
Also, developments in genetic engineering are supporting the optimization currently popular platforms to provide better expression of quality product. Metabolic engineering can improve peak densities, culture duration, cell-specific productivity, and product quality.
Next, there are the biological platforms employed. While CHO has been the most popular, other platforms are in consideration. Human cell lines provide human-like PT modifications. E.coli is popular due to its low cost, understood metabolism and genetics, as well as growth rate and productivity. Yeast, such as Pichea pastoris (P. pastoris) and Saccharomyces cerevisiae (S. cerevisiae) are gaining traction in recombinant biopharmaceutical production. Finally, transgenic plants present such advantages as low cost, safety, ease of scale-up, stability, and ability to produce N-glycosylated proteins.
Improvements in existing process trains are being more often considered, especially when synergies or serendipities appear. Examples of this include employing perfusion in amplifying seed train cell densities. This can either shorten the seed train footprint and duration by eliminating an entire n-x step or support higher peak cell densities because a higher-density seeding can shorten the metabolic activity of the culture to achieve peak densities. Benefits of simplification include reducing the time required per batch, increasing the potential batches per month or year.
Continuous bioprocesses can often provide more cost-effective options. Cost-savings include reduced classified space requirements, number of centrifuges, and reductions in downstream consumables. Intensification benefits include increasing the process productivity per time and footprint. As the operation can extend over many weeks (or even months), eliminating entire process changeover steps. Such further advances as “intensified perfusion” processes can dramatically increase the productivity. Optimizing a perfusion process involves media composition, cell density choice, feeding strategy, cultivation time, perfusion rate, and culture duration.
Culture medium or feeding solution optimization in any mode can increase productivity. Primary factors here include establishing culture longevity over short-lived peaks and product secretion over culture mass expansion. Optimal composition of a culture medium for the same cell line employed in batch, fed-batch, perfusion-enhanced fed-batch and perfusion-based continuous operations can vary greatly.
“Digital” approaches in biomanufacturing are varied, and the growing applications are promising to become major contributors to intensification efforts. Demonstrating the power of these “4.0” approaches in biopharma is how Moderna, a developer of messenger RNA (mRNA) based therapeutics, won the 2019 ISPE Facility of the Future award. This highly automated enterprise established the first human tests to suppress epidemic COVID-19 by delivering a batch of clinical vaccine to the National Institute of Allergy and Infectious Diseases (NIAID) 42 days from sequence identification.
Data sources, transmission, storage, and governance in biomanufacturing are maturing at a rapid pace. Sources of massive amounts of process data include new probes, sampling technologies, monitoring instrumentation and analytical procedure types, as well as high-throughput, multiplexed analytics.
Superior process control systems often employ supervisory control and data acquisition (SCADA). Model-based adaptive control now supports closed-loop bioreactor control for many variables and actuate dynamic changes in output variables. Both bioreactor type and process control are now important factors to consider in bioprocess intensification.
Digital twins are virtual models of physical systems or processes that convert a physical instrument and related activity to an in-silico model. They provide a digital transformation of the process to support the analysis, optimization, prediction, and even control of a manufacturing process in real time. In the near future we envision mechanistic modeling and AI supported machine learning employed in a digital twin to support a true “factory of the future”.
Artificial intelligence is a simulation of human intelligence in machines. It provides the ability to “rationalize” and come up with novel solutions to complex problems with rather undigested data. AI will soon be making appropriate bioprocess development/prediction/control decisions using advanced monitoring, big data processing capabilities and industrial connectivity.
Some improvements in process monitoring involve the means to monitor entirely new process parameters, while others apply to the monitoring of existing parameters. Both can support the intensification of a process. Improvements are occurring in many arenas, such as basis of the sensing, e.g., electrochemical, capacitance, surface plasmon resonance, mass-based, optical, thermistor or piezoelectric-based. So-called “biosensors” exist where surrogate measurements are converted to a functional value in silico. There is the growing maturity of automated sampling and distribution of bioreactor cultures. Finally, brand new instrumentation that provide rapid primary and secondary metabolite information are appearing.
Some designate assemblies of these initiatives as “computer-aided biology” (CAB), or an orchestration of tools replacing human activity in research. CAB divides activities into two realms: the digital and the physical. The digital, introduced above, includes advances from more powerful hardware to AI. The physical includes systems supporting the automated incorporation advanced experimental designs into actual laboratory experiments.
Selecting the best recombinant clone has historically emphasized small-scale cultures in microtiter plates, test tubes, and culture flasks looking for high product accumulation. Newer techniques have allowed including such criteria in a culture behavior and robustness in the production media, in the actual production mode envisioned (such as continuous), in scale-down conditions, and using a product quality profile.
Cloning, cell-line development and gene editing are being greatly advanced through such radically new technologies as microfluidics . One new approach uses a “laser tweezers” type of technology that directs millions of light-actuated pixels to move individual cells so they can be isolated, cultured, assayed and exported. Suppliers describe software algorithms that automatically identify single cells and then gently direct them into one of over 14,000 wells in a microfluidic chip in less than 30 minutes.
Single-use bioreactors have seen steady improvements the such things as impeller design, providing improved mixing and mass transfer, as well as re-design of the tank and sparge apparatus. Single-use upstream processing results in faster product-to-market, less capital and operating costs, smaller footprint, and greater flexibility. Loss due to contamination is reduced, but the most significant benefits in general are faster and easier tank preparation and turnaround times‘ lower capital and utility costs, and reduced concern for cross-contamination.
Prefabricated and modular facilities, podular suites and modular operations units are maybe the newest and most financially significant BI innovation. They can reduce footprint, capital expense, time-to-market, service needs, and operating expenses. Facilities composed of prefabricated and modular units feature rapid build and deployment of cGMP compliant design, materials, space allocation, workflow, equipment, utility and HVAC for both research and manufacturing. Prefabricated podular cleanrooms can be rapidly installed in unclassified (gray space) environments. Some prefabricated, modular, controlled-environment chambers combine principles of process intensification, automation and in-line chaining in self-contained ISO 14644-1 classified operating modules. They provide upstream, downstream, and aliquoting capacity that drastically reduce facility footprint, capital and operational expenditures, and are especially suited for such applications as viral vaccines, gene therapies, and oncolytic viruses.
That’s quite a lot. Are such tools available at this time to manufacturers and contractors?
As with other rapidly growing technologies, there is a difference between what exists in the university or supplier’s lab, and what is commercially available. But there is a steady progression of approaches from research to commercialized application. For example, my company, Cytiva (formerly GE Healthcare Bioprocess), is releasing products that support process intensification. One is a new perfusion-based large-scale bioreactor and the other is Fibro, a recently launched rapid cycling fiber-based chromatography platform.
Some firms advertise on-line optimal experimental design in robotic fed-batch cultivation facilities. Here, integrated frameworks provide real-time optimization of parallel nonlinear dynamic processes. The intent is to reduce the effort in providing precise estimates of a growth model’s parameter sets. Such a system uses two fully-automated liquid handling robots. One contains eight mini-bioreactors while the other is used for automated at-line analysis supporting the immediate use of the available data. It is powerful in allowing on-line, real-time re-design of experiments through parallel, concurrent re-computation of the experiment.
A fully automated ”GMP-in-a-box” concept for patient-scale cell therapy manufacturing is in final stages of development. This closed, automated, highly flexible cell therapy manufacturing platform employs single-use, highly customizable cassettes. It supports fully-enclosed, end-to-end manufacturing of cell therapy products with key unit operations, including isolation, activation, transduction, expansion and harvest. Technical features include monitoring and control of temperature and gases, pH and DO, and information logging and control with EBRs .
There are four manufacturers of automated bioreactor sampler/distribution systems, each with its own power and features.
And, of course, Cytiva is the producer of the KUBio modular biomanufacturing environments. These turnkey biomanufacturing solutions for cGMP production provide the intensification features described above for prefabricated and modular facilities.
How does this factor into the growing concern for Biomanufacturing Sustainability goals?
We often think of green initiatives as bringing added effort delay or expense. But surprisingly, they can sometimes actually provide actual cost savings and reduction in effort. Many process intensification initiatives not only increase productivity, but actually reduce environmental stress. For example, perfusion culture in the seed train can allow for the skipping of an entire culture expansion cycle, saving cost. This not only reduces expenses, but also saves an entire cycle’s worth of single-use materials. Also, a platform clone engineered to secrete more product can not only raise productivity per liter of media, but per piece of single-use material or unit of energy—and thereby reduce the processes direct environmental footprint.
Header Image Courtesy of www.davetang.org
About the Interviewee:
William Whitford, Life Science Strategic Solutions Leader, DPS Group, (formerly Strategic Solutions Leader at Cytiva)
Bill Whitford joined DPS Group as the Life Science Strategic Solutions Leader. Here he will assist in developing creative strategies supporting the manufacturing of both classical and innovative biotherapeutics.
Bill began his carrier as an R&D Leader, commercializing over 40 distinct products supporting biomedicine and biomanufacturing. Applications ranged from assisted reproduction to the culture of animal cells in protein biological and vaccine production.
Most recently Bill has been a thought leader identifying burgeoning biomedical products and processes. An invited lecturer at international conferences, he has published over 300 articles, book chapters, and patents in bioproduction; is a regular presenter at international conventions; and is an instructor in biomanufacturing.