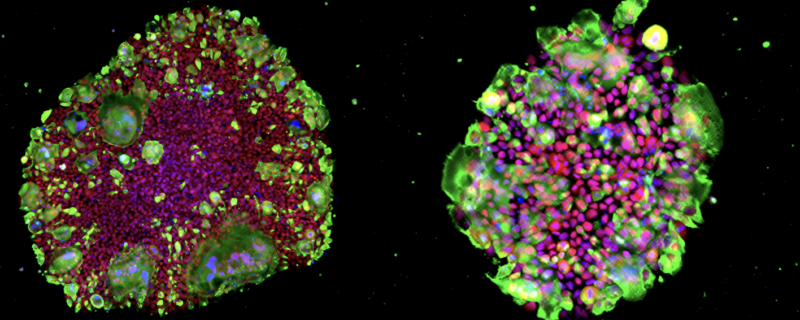
Culture Techniques for Expanding a Uniform Set of iPSC for Drug Discovery and Therapeutic Use
Application of iPSCs
In biomedical research, induced pluripotent stem cell (iPSC) technology represents a transformative milestone, offering self-renewal and differentiation capabilities without the ethical concerns of embryonic stem cells. This has led to Human iPSCs proving an invaluable resource for basic research, drug discovery, and regenerative medicine.
Prior to the first generation of iPSCs by Professor Yamanaka in 2006, patient-specific and replicative disease human models were limited, which posed significant challenges to the translation of research into therapeutic developments. The emergence of iPSCs provides an opportunity for autologous cell therapies that were previously restricted by immunological and ethical obstacles. However, despite this notable progress in cell and gene therapies, challenges have arisen due to the resource-intensive, time-consuming, and variable nature of iPSC generation. The development of scalable and reproducible production of iPSCs and their differentiated progeny is a major requirement before iPSCs can be clinically implemented and useful.
Importance of iPSC Expansion
When using iPSCs for Drug Discovery and therapeutic applications, ensuring the generation of a homogenous set of iPSCs is key. The absence of uniformity in iPSC cultures can severely compromise the quality and quantity of data generated. Therefore, it is important to develop a reproducible process of iPSC production that prioritizes quality and scalability.
Central to this is the development of a reproducible protocol for producing a working cell bank (WCB) of iPSCs. WCBs are used to maintain a consistent supply of cells. Throughout the process of creating a WCB, careful consideration must be given to several factors such as genetic stability, optimal culture conditions, and cryopreservation techniques to ensure reproducibility of results and consistent product quality. Understanding these factors is pivotal for ensuring the integrity and utility of iPSC- application.
Maintaining Genetic Stability
The genetic stability of iPSC cultures hinges on various cell culture conditions, including media composition, pH levels, oxygen concentrations, and substrate stiffness. For instance, acidic pH impedes glucose consumption, escalating cell death, while the presence of Reactive Oxygen Species correlates with genetic alterations in stem cells (Wilmes, 2017 & Thompson 2020). Genetic mutation in cell populations results from two steps: mutation (the step whereby a cell acquires a mutation) and selection (the step whereby the mutated cell expands rapidly by the acquisition of survival advantage). Suppressing the selection of mutated cells is also an effective approach to maintaining genetic stability in cell populations (Jason 2020).
Genetic instability, which often reveals hidden challenges, becomes apparent during later stages of stem cell differentiation. Alterations in the resistance of apoptotic genes can yield “false positive” experimental results, emphasizing the need for comprehensive measures like g banding and next-generation sequencing to identify genetic changes, depending on the application. Although genomic analysis by next-generation sequencing provides information at the single nucleotide level, the impact of single nucleotide variants on in-vivo phenotype is not fully understood, and appropriate testing methods and criteria need to be selected (Takako 2022 and Merkle 2017). Additionally, the choice of ancillary materials, such as StemFit® media, proves crucial for iPSC expansion, offering high rates with optimized genetic stability in creating an ideal WCB with iPSCs.
Importance of Cell Culture Conditions
The importance of chemically defined cell culture conditions for iPSCs lies in their ability to provide a precisely controlled and reproducible environment for cell growth and maintenance. Unlike undefined or serum-containing media, chemically defined conditions involve using well-defined and purified components, eliminating variability and batch-to-batch inconsistencies. This level of precision is crucial for iPSCs, as they are sensitive to their microenvironment (Ozawa 2023).
Chemically defined culture conditions are exemplified by cutting edge products such as StemFit® culture media which offer researchers greater control over factors such as media composition, growth factors, and substrate properties, ensuring a more standardized and predictable cell culture environment. This standardization not only enhances the reproducibility of experiments but also minimizes the risk of introducing unwanted variables that could impact the genetic stability and differentiation potential of iPSCs. In addition, chemically defined culture conditions align with current trends in regulatory requirements in bioprocessing and clinical applications, making them essential for advancing the reliability and translational potential of iPSC-based therapies and research.
Managing Single Cell Expansion
Single cell expansion is a rising and popular method for producing consistent iPSC WCBs. The biggest advantage of single cell expansion is the ability to seed a fixed number of uniform single cells, which allows for highly reproducible culture, – in contrast to clumping where it is difficult to strictly control the cultural condition.
In some cases, single cell expansion when not done correctly can cause complete dissociation of iPSCs leading to apoptosis and cell death. Therefore, to counter this issue there are several factors to consider (Ozawa 2023).
Using iMatrix-511 laminin E8 fragments facilitates single-cell expansion by providing extracellular matrix (ECM) support, promoting cell survival through fast attachment of cells and appropriate migration. Another method to consider is to use small molecules like ROCK inhibitors which enhance cell survival, cloning efficiency, and culture health through preventing apoptosis (Watanabe 2007). The use of gentle dissociation solutions, such as Detachin™, also helps to avoid cell loss during harvesting and passaging, cells should be primed for detachment and rinsed without completely detaching from the surface enhancing maximum cell viability. Maintaining an appropriate culture density becomes imperative in ensuring the optimal conditions for iPSC cultures. It is advisable to passage cultures before reaching 75% confluency to avert the risk of differentiation induced by an overly populated environment. This practice aligns with the broader goal of promoting a controlled and balanced cell growth environment, mitigating the potential negative effects associated with excessive cell density.
The Impact of Cryopreservation
Cryopreservation plays a pivotal role in preserving biological materials, ranging from cells to tissues and organoids. This process halts metabolic activity and preserves the structural integrity of these biological entities, allowing them to be stored for extended periods without degradation.
Past methods for preserving cells with vitrification have created issues from its rapid cooling rates which can damage fragile stem cells. To solve this issue researchers have pivoted to using a basal media with an addition of 10% DMSO. This method has also been shown to be problematic due to its low reproducibility and changes in cellular morphology post freeze thaw. Thus, researchers have looked at standardized cryopreservation media for higher yield, minimal disruption in stem cell morphology, and reproducibility.
To maximize the efforts that were produced throughout the culturing procedure – the cryopreservation protocol should also include post-freeze instructions. Integration of advanced cryoprotective solutions like Stem-CELLBANKER® further enhances key stem cell attributes post freeze thaw. Cell recovery can be maximized to 90+%. Key genetic markers (OCT4, SOX2, and NANOG) have also been shown to be conserved post freeze thaw.
Throughout stem cell applications it is imperative to cryopreserve cells. When considering cryopreservation within your own, consistent and repeatable protocols must be developed to ensure optimal results.
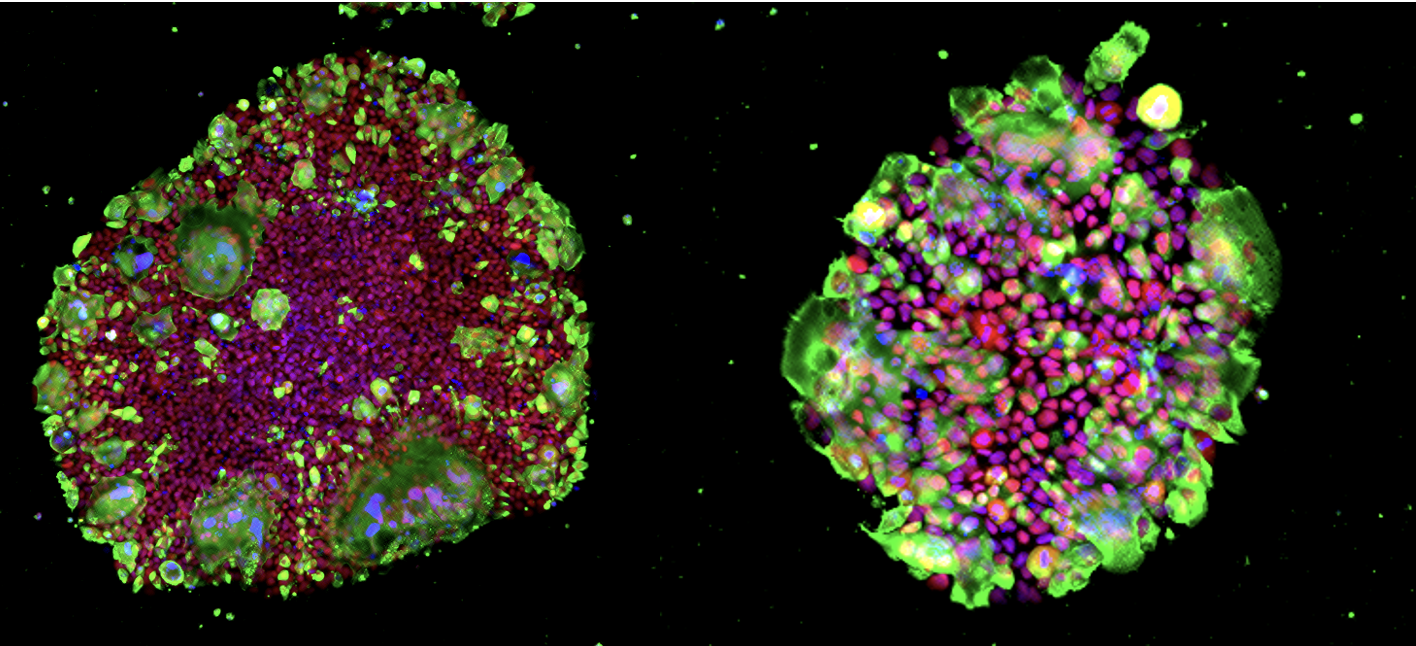
Green: SARS-CoV-2 N protein, Red: OCT3/4, Blue: DAPI. Images courtesy of Kazuo Takayama (CiRA, Kyoto University, Japan)
Future Prospect in 3D Suspension
Current challenges remain in developing iPSC cultures. It has been found that human clinical cell therapy requires 108-1010 clinical-grade stem cells cultured under cGMP conditions, while commercial-scale manufacturing requires approximately 1011 -1014 cells (Pigeau 2018). Under current 2D expansion methods of iPSCs this would create a barrier similar to the one seen with autologous therapy and cost. This cost is mainly attributed to the material and handling cost associated with many flasks used during the development of WCBs. To overcome this barrier 3D suspension cultures could be a possibility. 3D suspension cultures in bioreactors allow for a higher quantity of cells, less media consumption, and reduced handling. Thus, contributing to effective cost savings for further development of iPSC use.
Challenges persist in the advancement of 3D cultures due to uncontrolled spheroid aggregation and cell shear stress, leading to issues like cell growth inhibition, apoptosis, and variability in quality (Kinney 2011). Transitioning common techniques used in 2D environments, such as coating plates with vitronectin or laminins, to 3D suspension cultures is not feasible. In the 3D context, novel methods are needed to establish an effective extracellular matrix (ECM) conducive to stem cell production. Laminin E8 fragments offer a solution by enabling the incorporation of an ECM component into suspension cultures to create an ECM layer.
Conclusion
iPSC cultures have a vast collection of factors that contribute to the downstream viability of your WCBs. Monitoring genetic stability and identifying genetic instability through rigorous quality control can be imperative to identifying adequate iPSC cultures. Technical culture aspects such as using animal origin free (AOF) defined media and culture conditions with single cell expansion can create consistency in your cultures. Another factor identified outside the culturing protocol is the cryopreservation of iPSCs. This was also found to have a profound effect on iPSCs future viability post thaw. Lastly, with this new scientific modality, there are still many hurdles to overcome in culturing techniques and quality control processes to realize the advantages of iPSCs.
Find out how AMSBIO can support your stem cell research, https://www.amsbio.com/stem-
We are also keen to learn more about stem cell research: we’d love to hear from you at info@amsbio.com
References
Halliwell, J., Barbaric, I. & Andrews, P.W. Acquired genetic changes in human pluripotent stem cells: origins and consequences. Nat Rev Mol Cell Biol 21, 715–728 (2020). https://doi.org/10.1038/s41580-020-00292-z
Kinney MA, Sargent CY, McDevitt TC. The multiparametric effects of hydrodynamic environments on stem cell culture. Tissue Eng Part B Rev. 2011;17(4):249–262. doi: 10.1089/ten.teb.2011.0040
Merkle F, Ghosh, S., Kamitaki, N. et al. Human pluripotent stem cells recurrentla y acquire and expand dominant negative P53 mutations. Nature 545, 229–233 (2017). https://doi.org/10.1038/nature22312
Ozawa H, Matsumoto T, Nakagawa M. et al. Culturing human pluripotent stem cells for regenerative medicine. Expert Opinion on Biological Therapy. 2023, VOL. 23, NO. 6, 479–489. https://doi.org/10.1080/14712598.2023.2225701
Pigeau GM, Csaszar E, Dulgar-Tulloch A. Commercial scale manufacturing of allogeneic cell therapy. Front Med. 2018;5(AUG). doi: 10.3389/fmed.2018.00233
Thompson M, Mei SHJ, Wolfe D, Champagne J, Fergusson D, Stewart DJ, Sullivan KJ, Doxtator E, Lalu M, English SW, Granton J, Hutton B, Marshall J, Maybee A, Walley KR, Santos CD, Winston B, McIntyre L. Cell therapy with intravascular administration of mesenchymal stromal cells continues to appear safe: An updated systematic review and meta-analysis. EClinicalMedicine. 2020 Jan 17;19:100249. doi: 10.1016/j.eclinm.2019.100249.
Watanabe K, Ueno M, Kamiya D, et al. A ROCK inhibitor permits survival of dissociated human embryonic stem cells. Nat Biotechnol. 2007;25(6):681–686. doi: 10.1038/nbt1310
Wilmes A, Rauch C, Carta G, Kern G, Meier F, Posch W, Wilflingseder D, Armstrong L, Lako M, Beilmann M, Gstraunthaler G, Jennings P. et al. Towards optimisation of induced pluripotent cell culture: Extracellular acidification results in growth arrest of iPSC prior to nutrient exhaustion. Toxicology in vitro, 445-454 (2017). https://doi.org/10.1016/j.tiv.2017.07.023.
Yamamoto T, Sato Y, Yasuda S, Shikamura M, Tamura T, Takenaka C, Takasu N, Nomura M, Dohi H, Takahashi M, Mandai M, Kanemura Y, Nakamura M, Okano H, Kawamata S. Correlation Between Genetic Abnormalities in Induced Pluripotent Stem Cell-Derivatives and Abnormal Tissue Formation in Tumorigenicity Tests. Stem Cells Transl Med. 2022 May 27;11(5):527-538. doi: 10.1093/stcltm/szac014. PMID: 35445254; PMCID: PMC9154342.