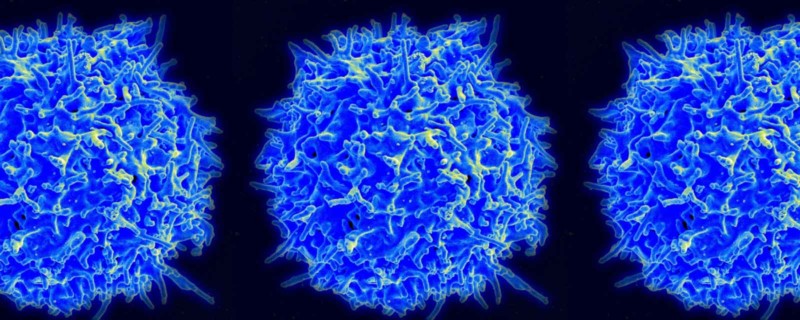
The Evolution of Cell Therapy Manufacturing – Early Days and Present-Day Procedures
While often casually mentioned in a single phrase, cell therapy (CT) actually refers to quite a wide spectrum of medical treatments. In the EU, they can be referred to as cell based medicinal products (CBMP), a type of advanced therapy medicinal product (ATMP). In the US, CBER continues to clarify such concepts as human cellular and tissue-based products (HCT/Ps) and cell-therapy medicinal products (CBMPs), with respect to minimal manipulation, other component combination, systemic effects, and metabolic activity dependents.
Distinctions in the therapies include autologous vs. allogeneic approaches; the cell type employed; any activation, transduction, or engineering required; the mode of cell culture; and the therapeutic indication. We are all aware of the T cells and many varieties of stem cells used in therapies. But in fact, many cells of innate or acquired immunity known for phagocytic activity, antigen presentation capability, flexible phenotype, or tissue migration are now being addressed as therapeutic agents. Cells adopted from peripheral blood alone include T, natural killer (NK), induced pluripotent stem (iPS), macrophage, gamma delta T(g∂T), Marrow-Infiltrating lymphocytes (MILs) and Tumor-Infiltrating Lymphocytes (TILs) and hematopoietic or mesenchymal stem cells.
Some have been engineered by such means as virus transformation or CRISPR-Cas9 toward several therapeutic technologies, such as chimeric antigen receptor (CAR) T Cells and now even dimeric antigen receptor (DAR) T Cells, and are being applied in the treatment of several inflammatory and proliferative diseases. In general, the actual manufacturing of cell therapies, while at a smaller scale, is a more complex and labor-intensive process than the production of protein biologicals such as MAbs.
In this two part series, we examine the evolution of cell therapy manufacturing, including how cell therapy got its start and early cell processing procedures. From there, we look at the current state of cell therapy manufacturing and present-day methodologies. In part two, we will discuss opportunities to improve on current processes and examine future cell therapy manufacturing that will carry the industry into the future.
Early days
It is not surprising that the transfer of cell therapy processes to commercial-scale manufacturing began rather awkwardly. First, procedures developed for the culture, modification and expansion of cells for even such advanced therapies as stem and CAR-T cells began (surprisingly) in the late 1980s when technologies were quite primitive by today’s standards. These early procedures were characterized by hand-labeling, manual dispensing, paper-based tracking, as well as limited analytics and often poorly employed data pools. Because of this “the process is the product” mentality inevitably slowed the incorporation of newer engineering and cell culture procedures.
For example, the processes for generation of TIL used in Rosenberg’s earlier adoptive transfer therapies were characterized by in-house produced materials, manipulated in biosafety cabinets of laboratories in highly specialized medical centers. Here, non-cryopreserved patient’s tumor biopsies were often diced, incubated in an enzyme cocktail, and the harvested slurry filtered through a wire mesh to remove tissue chunks. TIL cultures were established from this filtrate by isolation in a step Ficoll gradient followed by manual passaging in 24-well plates with IL-2. TILs were then expanded in T-flasks or plastic bags, sometimes with conditioned cell culture supernatant or co-culture with irradiated feeder cells [ 1 ].
Current State
While bone marrow transplantation might be considered the most successful cell therapy, of the newer cell-based immunotherapies in development the furthest advanced in clinical application are stem and CAR-T. About a decade ago, in CAR-T we began to see the emergence of not only new antigen receptor engineering technologies, but of the cell types recruited in CAR therapies. Concurrently, we have seen adoption of significant developments in cell processing equipment, isolation and expansion approaches, analysis and cryopreservation techniques.
Some current processes are possible bedside, at the point of medical care− but many currently favor a centralized manufacturing approach where the apheresis or biopsied material is shipped fresh or frozen to a centralized manufacturing site. Here the cell product is manufactured, cryopreserved and shipped frozen to the clinic for infusion into the patient [ 2 ].
Aspects of both the manufacturing process and facilities for many cell therapies are quite similar to those of other biologicals [ Figure 1, 2 ]. For example, the expansion of cells in a bioreactor for such allogeneic cell therapies as immunoprivileged MSCs is quite like that of mammalian cell culture for a vaccine and may eventually require a similar scale of production[ 3 ]. However, other aspects are quite different. One characteristic of cell-based therapies is that the final product cannot be filter sterilized, as it is in mAb or enzyme manufacturing. As individual cell therapies have evolved, considerations for their manufacturing have become more diverse. The requirements in segregation, containment, and material flow through a facility can result in significant design variation. For example, the segregation of multiple patient samples or infectious materials can determine isolation requirements.
Figure 1. General biomanufacturing facility requirements
- Gowning rooms: Personal Airlocks (PAL) and Material Airlocks (MAL)
- ISO classifications and cGMP compliance
- HVAC, air supply and airflow patterns
- Temperature and humidity control
- Environmental monitoring
- Aseptic processing requirements
- Contaminant and particulate control
- Critical utilities (and redundant or backup electrical)
- Process utilities (Water for injection, CO2, compressed dry air, etc.)
- Size of the facility (dependent upon functions required and outsourcing)
- Autologous or allogeneic and their associated production process layout
- Specialized equipment required (e.g., bioreactor, centrifuge, cryopreservation)
Figure 2. Equipment often employed in cell therapies
- CO2, humidified cell-culture incubators
- Antibody based cell separation devices
- For-purpose cell handling/culture/production system
- Biosafety cabinets and/or aseptic containment isolators
- qPCR, flow-cytometer, or ELISA/PAGE
- Centrifuge to support leukocyte separation
- Laboratory microscope
- Gamma irradiator
- Flow cytometer
The manufacturing of CBMPs to support any of these therapies can require such operational features as specialized receiving and shipping, advanced chain of custody and track/trace, special regulatory considerations, unique automated single-use systems, open or closed system processing, cryopreservation, large-scale or multiple small-scale cell culture operations. It now can employ ballroom, process automation, unique post-culture or downstream processing and advanced laboratory testing. Operations suites are designed to handle required cleaning and disinfection, and their environment classifications follow both the nature of the product and the process equipment employed. CT facility design requires careful reckoning of science-based safety and regulatory standards. For example, in establishing suite specifications, closed and automated processes permit reduced suite classification as do processes operated in aseptic containment isolators.
Over the past few years there has been an increase in the number of cell and gene therapy products licensed and approved by CBER’s OTAT (Office of Tissues and Advanced Therapies) and the EMA, as well as a great expansion in the number of submissions under review. While there are some rather bold initiatives in commercial-scale manufacturing proposed, for many there often remains a conservative approach in process scale-up, suite design and facility architecture. Below is a glimpse at some categories of the processes are they are currently operated.
Sample reception
For autologous therapies the reception, cataloging, and governance of hundreds of unique shipments of patients’ cells requires dedicated processes and facilities. The first step in processing such therapies is moving samples from contaminated shipping containers in non-controlled environments to sterile equipment in controlled environments. In these early days of operation we are not considering enormous volumes, and even 2,000 batches per year equates to only a few batches per day− although this is expected to soon change.
Culture media preparation
Facilities for the use of liquid vs. powdered media and buffers are very different. Powdered medium for larger-scale culture requires suites capable of handling the powder formulation and filtration. Also, space is required for QC testing, transfer, and dispensing of sterile fluids for the closed and/or integrated systems used in production. If liquid products in scale-appropriate containers are employed (which is common for manufacturing at current scales), none of the above is required, but sufficient refrigerated space for their storage and organization must be designed.
Vector production
For processes requiring vectors, there are many options currently available in gene vector choice, production methods, and site of manufacture. They include various sources of naked DNA and nanostructure, electroporation, microfluidics, and recombinant virus vectors produced in mammalian and insect cells. Few CT sponsors have the capacity, expertise, or resources to manufacture viral vectors, which is why there is a growing market in their third-party contracting [ 4, 5 ]. Much work is being performed on multiply recombinant “producer” cell lines, yet work remains to be done in this field for practical success− and viral vectors remain the approach of choice. While many viral vectors / animal cell lines exist, each with their own strengths, for CT applications, lentiviral and AAV produced in HEK 293 are currently the most popular.
Facility requirements for viral vector production are essentially the same as some vaccine production, with the exception of exact scale, suite classifications, and downstream train configuration. Formal BSL classification for nanoparticle, adenovirus, lentivirus, and AAV virals vary. Factors in the BSL classification of a virus include 1) the family and sub-family of the virus, 2) any recombinant inserted genes, 3) the means of production (e.g., helper virus), and 4) any engineered attenuation. For example, individual activities with early generation lentivirus must be conducted under at least BSL-2, enhanced BSL-2 or BSL-3. AAV constructs can generally be handled at BSL-1; however if certain transgenes are included, or if a helper virus is used during the production, BSL-2 containment can be required. For these reasons, and to provide flexibility in accommodating changes in process or virus, many sponsors default to a higher BSL classification. Containment requirements and means are addressed by individual agencies, consortia, and committees, such as the Recombinant DNA Advisory Committee (RAC) of the NIH in such documents as “Biosafety Considerations for Research with Lentiviral Vectors” [ 6 ].
Most vectors in clinical trials are of viral origin, although some sponsors are now developing, e.g., transposon-based vectors delivered by synthetic targeted nanoparticles. Obviously, each system determines its own manufacturing process and requirements. Manufacture of many viral vectors had, up until recently, required adherent cell culture in multi-tray static plasticware, roller bottle, cubes, multilayer cell factories, microcarriers in stirred tank bioreactors, or scalable fixed-bed reactors [ 7 ]. But this is changing, and the majority of the cell lines used to generate viral vectors today are suspension adapted. Furthermore, requirements of sterility, reproducibility, and manufacturing staff safety are driving the development of semi-closed and semi-automated manufacturing processes.
While high titers of vector can be achieved using suspension adapted cell lines, there are challenges reported with transient transfection of suspension cells at scales >200L, and methods using suspension cells at larger scale have not been widely adopted for routine vector production.
Therapeutic Culture Expansion
The goal in cell expansion is a simplified, robust, GMP compliant approach. Steps vary between particular cell therapies, as well as between individual implementations of a particular therapy− and we can see distinct cell culture activities prescribed. For example, in a CAR-T process we see 1) culture of the selected apheresed patient cells to activate and transform, 2) expansion of the activated and transformed patient cells, and 3) in some cases, the co-culture of the expanded patient cell mass with irradiated antigen-expressing feeder cell lines.
It’s apparent that these multiple handling steps can become relatively complex. This presents at least two challenges to efficacy and reproducibility. First, because the primary patient cell samples are unique and highly variable in multiple parameters, the process must be dynamic and responsive to the characteristics and performance of that particular culture. Secondly, each step presents a separate risk of potential operator error. Sponsors and contracted partners are therefore working toward the development of more optimized and automated protocols and facilities. This will help reduce costs and error risk, while increasing standardization and reproducibility.
Some CT involve suspension (e.g., CAR-T) and some adherent cell culture (e.g., mesenchymal stem/stromal cells, fibroblasts, and myoblasts). The intricacies of working in either system have been greatly reviewed [ 8 ]. There is also a desire to intensify the process with perfusion-based culture systems.
In the large-scale (greater than a few liters or multi-layer flasks) culture of immunoprivileged cells for allogeneic approaches, we hope to see upstream suites and equipment familiar to those in protein biological manufacturing. Familiar methods of master and working cell stocks, culture expansion trains, large-scale culture and process monitoring control would be employed. In autologous cell therapies, the individual origin and characteristics of the patient-specific cells, and the very small-scale of culture (<20L) are the most significant differences. Finally, we now see the widespread adoption of commercially distributed semi-automated culture instrumentation [ Table 3 ].
Single-use system-based processes are common and employ sterile SU flasks, bottles, bags, tubing sets, filter trains, and even automated systems. These components are often bulky and therefore require dedicated storage space to avoid excessive stacking and associated risk of damage. A critical aspect of the overall manufacturing process contamination control strategy (CCS) will be areas for the handling, preparation, associated sanitization and inspection of components prior to and during assembly. For critical operations, e.g. drug product filling, preparation and assembly should take place in an environment of high classification, which may be in the different area to that of final operation. Where this is the case, the transfer of assembled components to the areas of use and the associated sanitization regimes (e.g., manual, VHP surface bio-decontamination airlocks) will critical and should be addressed in the CCS.
Table 3. Example commercialized CT equipment
Autolomous | integrated automated CGT data hub |
---|---|
Cellaris | Cell Shuttle “factory-in-a-box” system |
Comercer | Aseptic process containment technologies |
Cytiva | Sepax cell processing / separation system Xuri Cell Expansion System |
Fresenius Kabi | Lovo Automated Cell Processing System |
Lonza | Octane Cocoon |
Miltenyi Biotech | CliniMACS Plus separation system CliniMACS Prodigy cell processing system |
Terumo | Reveos, COBE 2991 |
ThermoFisher | Nunc Cell Factory Herasafe biosafety cabinet |
Wilson Wolf | G-Rex static culture systems |
Analytics
The quantitation and analysis of cells, viruses and nucleic acids are required for many platforms, and often employ rather new types of equipment. However, other than potentially having an additional safety classification (i.e., BSL-2) suites supporting this function generally have few unique or remarkable design attributes. Incoming raw material QC processes are exactly like those of protein biologicals. In-process and 21 CFR final product QC and release testing for a living cell product can be quite unique from a small molecule or protein biological and involve establishing values for such production parameters as cell number and viability, and such product related impurity values vector competency, cellular CD marker, and CAR expression. But, other than footprint, even the commonly employed qPCR, flowcytometric, or ELISA/PAGE analysis poses no incremental facility or suite design or service challenges. Also, means of the characterization and quantitation of viral vectors has progressed substantially [ 9 ].
Cryopreservation and shipping
The transfer of CT cellular materials and products often require cryopreservation. Both the harvested patient’s cells shipped to the manufacturing site, as well as the manipulated pharmaceutical ingredient returned to the clinic, require the same kind of seamless transportation. Integrated, single-use freeze–thaw systems can provide efficiency and robustness where there may be very little material or time available for a second try. For large-scale allogeneic cell processing, facilities supporting the semi-automated aliquoting of product are established. For autologous therapies, this involves the highly controlled dispensing and labeling into vials or cryobags. It can be difficult to automate the filling procedure for unique batches of individual volume, or other characteristics, into individual cryo-bags.
Automation, Semi-automation, and Robotics
Autologous therapies, especially traditionally, have required many skilled and technically experienced personal. Frequent training is required as many manipulations have been performed in open systems which are prone to human error. The availability of commercialized semi-automated systems and even robotics in CTs have greatly advanced their safety and efficiency [Table 3].
Facility Flexibility
Most new builds incorporate proprietary modular cleanroom systems. Many modular designs include wall and ceiling systems with flush-fitting panels supporting easy assembly and disassembly as well as required cleaning and disinfection. Working environment classifications follow both the nature of the product and the process equipment employed. Modern facility design requires careful reckoning of science-based safety and regulatory standards. Closed and automated processes require significantly reduced suite classification as do processes operated in aseptic containment isolators.
Conclusion
Cell therapy manufacturing processes originated decades ago from early procedures, employing what we see now as rather primitive technologies and equipment. Current approaches are somewhat disparate, with mature sponsors adopting some of the newest approaches to cell isolation, engineering, analysis, and semi-automated culture. We are in a maturing state of many filings, some significant approvals, and the promise of exciting new production technologies and facility design options.
Stay tuned for Part II of our discussion, The Evolution of Cell Therapy Manufacturing – Opportunities for Advancement and Future Directions
Featured image credit: Flickr user NIAID
About the Author
William Whitford, Life Science Strategic Solutions Leader, DPS Group
Bill Whitford is the Life Science Strategic Solutions Leader for DPS Group, a global consulting, engineering and construction management company. He assists in developing creative strategies supporting the manufacturing of both classical and innovative biotherapeutics. Bill began his carrier as an R&D Leader, commercializing over 40 distinct products supporting biomedicine and biomanufacturing. Most recently Bill has been a thought leader identifying burgeoning biomedical products and processes. Bill has published over 300 articles, book chapters, and patents in bioproduction; is a regular presenter at international conventions; and is an instructor in biomanufacturing.