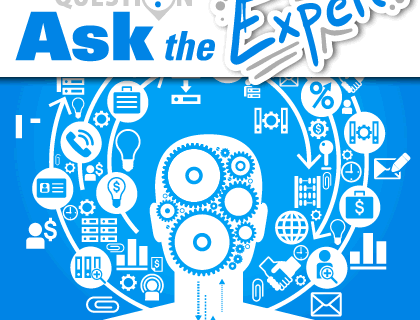
Implementation of Continuous Manufacturing Strategies for Protein Therapeutics
Company: WuXi Biologics
Job Title: Vice President, Cell Culture Process Development
Answers by: Hang Zhou, Ph.D., Director of Cell Culture Process Development, WuXi Biologics & Sherry Gu, Ph.D, Vice President of CMC Management, WuXi Biologics
Introduction
There continues to be growing interest in continuous processing of biological therapeutics with the primary driver being reduction of drug costs through more efficient manufacturing processes. Together, drug developers, contract manufacturing organizations and process equipment suppliers continue to make significant advancements towards the goal of making continuous biologics manufacturing a reality from the initiation of cell culture to the production of bulk drug substance. Compared to traditional batch technologies, continuous bioprocessing can offer more flexibility and in some cases improve product quality in addition to lowering the costs of production. Continuous cell culture technologies can be used in the manufacturing of both stable and unstable biological molecules. The US FDA has been supportive of the implementation of continuous manufacturing using a science- and risk-based approach.
This week WuXi Biologics’ offer Hang Zhou, Ph.D, Director of Cell Culture Process Development and Sherry Gu, Ph.D, Vice President of CMC Management as two industry experts in applied continuous manufacturing approaches to address any questions you may have related to the adaptation or implementation of continuous manufacturing strategies for protein therapeutics. WuXi Biologics has developed a continuous processing platform for biologics manufacturing using single-use bioreactors and have been successful in the development of multiple continuous unit operation steps both at small development scale and for large-scale GMP manufacture (1,000 L perfusion cell culture).
Question 1
For lot release, what do you consider a lot?
In the past there was concern over definition of a batch or lot but it is no longer an issue. A batch from a continuous process can be defined by batch duration and/or certain product amount. For early phase clinical production, one perfusion culture run is usually released as one lot – thus, harvest collections from different time points are processed to a stable intermediate and pooled later (after appropriate in process control testing is completed) to complete the rest of purification and release as one batch. For some long duration perfusion culture with good steady state and high productivity, it is feasible to split the product to multiple lots for release. In addition, multiple parallel, staggered or successive perfusion runs could be combined at a certain defined stage prior to drug substance final production and released as one lot.
Question 2
Do you run continuous downstream too? How do you connect your upstream and downstream?
Yes. There are various advantages to continuous downstream manufacture. It can be implemented together with continuous upstream to realize an integrated continuous process. For fed-batch culture, continuous downstream allows lower one-time resin expense due to use of smaller sized columns and higher resin utilization efficiency. The critical technologies that allow for continuous downstream processing are multi-column chromatography systems and single-pass Tangential Flow Filtration (TFF). Although full continuous processing is the ultimate goal, the most cost-effective application is to develop a continuous product capture step. We have successfully established continuous product capture using a multi-column chromatography system at bench-top scale and it is also working on the scaled-up process.
The connection between continuous upstream and downstream is quite straight forward if hollow fiber-based cell separation systems are used for perfusion cell culture and harvest - otherwise, additional clarification steps are required. Single-pass TFF can be used as an option to concentrate perfusion cell culture harvests before the product capture step if titers are too low. And intermediate storage or break tanks can be used as optional steps to ensure operational robustness.
Question 3
What product limits have you found with continuous with either product type or demand?
We don’t see many product related limits when using a continuous process scheme compared to a traditional fed-batch process. In fact, continuous manufacturing can accommodate a wide variety of molecules - including mAbs, fusion proteins, growth factors, recombinant enzymes, etc. - many of which could not be produced in other cell culture modes (such as fed-batch) due to product stability issues. The only limit we’ve observed is that some specific molecules may have product retention issues during continuous harvest when using certain types of hollow fiber filter (HFF)-based cell separation devices due to the proteins’ large molecule weight or other unique characteristics of the molecule. The challenge can usually be addressed by screening of different membrane materials and evaluation of membrane pore size and membrane area in the HFF system during process development. Alternatively, other cell retention devices with different mechanisms of action can be also considered, like those based on gravitation (inclined cell settlers, centrifugation) or acoustic resonance systems.
In addition, high productivity can be achieved with a reduced manufacturing footprint compared to fed-batch systems and the facility can be easily scaled-out to manufacture large quantities of product with reduced cost. For those products with relatively low demand, utilizing continuous processing can still be cost-effective because utilizing a well-developed and universal platform would require minimal process development and scale-up work.
Question 4
What are the main reasons that cause you to choose a continuous manufacturing vs. conventional?
There are several major drivers for implementing continuous bioprocessing. First of all, there are increasing needs for flexibility and speed to meet market demands. Continuous bioprocessing will allow the use of modular and smaller facilities with smaller and/or single-use bioreactors in order to meet rapidly changing and unpredictable market demands in diversified and segmented market places. Secondly, we expect that more low-volume biologics will need to be produced for personalized applications, in addition to historical demands for high volume biologics. Thirdly, both increased demands in emerging markets and introduction of biosimilars will increase competition and require lower COGs and therefore continuous processing could be used to address these various market factors. Thus, there are many advantages of continuous bioprocessing over conventional batch processing that should be considered including:
- A universal continuous platform for manufacturing could be developed for both stable and liable products
- Ability to achieve high cell concentration, cell viability and volumetric productivity
- Steady state operation that provides consistent product quality; low residence times
- Compatibility with the principles of QbD and manufacturability
- Multiple Operational advantages
- Simplified process flow & logistics, high operational flexibility
- Small equipment and facility footprint and lower initial capital investment
- Modular architecture providing fast technology transfer
- Minimal scale-up or potentially utilizing same scale for development, clinical and commercial manufacturing.
- Smaller facility footprint and modular nature allows for “scale-out” instead of “scale-up” manufacturing paradigm
Question 5
Have you applied PAT and do you find it is easier or more difficult in continuous? Are you able to monitor process conditions and make adjustments real time?
We’ve evaluated various PATs on continuous processes including auto-sampling systems and online probes and analyzers. Implementation of PAT is usually easier for continuous processes since we typically can develop and thus expect steady state conditions. We found PATs quite useful especially those that monitor key process parameters in real-time and others that help to identify and maintain steady state operations. One example is to use online biomass indicators (biomass probes or determination of Oxygen Uptake Rate derived from gas analyzers) to control the cell bleeding rate and therefore maintain a stable viable cell density. Another example is to use a pCO2 probe for adjusting the air sparge rate (for CO2 stripping) to keep the high pCO2 levels within the specified range - which is critical when working with high cell densities in a continuous cell culture process.
Question 6
Do you have to do much optimization on your perfusion culture?
For products (e.g.,monoclonal antibodies) that in general tend to behave similarly in production we typically do minimal process development work for early stage programs (e.g., prior to IND or for production of Phase I/II clinical trial material) but rather we leverage our well-established continuous “platform” process. For example, our platform comprises solid knowledge of our host cell line, media (in combination with the host cell line) and process control strategies. In addition, we know that the pre-characterized scale-down model process we use in development can usually be directly scaled up to clinical manufacturing scale thus minimizing the tech transfer efforts. Perfusion cell culture development could be costly and resource demanding so developing a platform process to streamline work for all future products is ideal. Of course there are needs for extensive process optimization in some cases, such as production of challenging molecules (e.g., unstable molecules), developing processes for biosimilars with strict quality requirements or for products entering later stage process development where the goal of reducing production costs and/or establishing more enhanced process robustness is critical. However, it should be noted that these same aforementioned process optimization requirements may also be required for fed-batch processes.
Question 7
Can you provide a timeline of your continuous manufacturing runs?
Timelines from cell expansion through continuous cell culture and potentially continuous downstream purification can vary widely pending on a multitude of factors that include actual product demand, the scale of bioreactors, the expression levels of the product and if continuous downstream is utilized, production timelines are impacted by pooling/holding strategy, the number of cycles required in continuous downstream purification which is dependent on the column matrix and size of column (amongst other factors) and certainly cost considerations all can impact the overall manufacturing timeline. We have conducted continuous cell culture for clinical production that ranges from 30 days to 60 days (and this range is consistent with many of the well-publicized industry continuous processes that manufacture commercial products). Thus, you can see that there is no standard timeline or general guideline for continuous manufacturing timelines and therefore it is a case-by-case situation.
Question 8
I know this is a complex question but can you give a high level about cost and if you see cost savings and where.
Continuous manufacturing is quite flexible and can be utilized at various production scales and configurations. Regardless of the case-by-case cost structure of every process, we will try to identify a few key factors that could significantly affect the cost of a continuous manufacturing process, in comparison to conventional processes. Generally speaking, continuous upstream processes can be cost effective when high volumetric productivity is achieved. Often this means a smaller (and less expensive) bioreactor can be used compared to fed-batch processes and therefore the facility or manufacturing suites footprint can be smaller as well and thus more efficient on a square foot perspective leading to cost saving in CAPEX and facility occupancy. In addition, the cost of disposable raw materials will be lower per product because fewer batches are required to achieve the same product demand levels and the longer duration of each batch manufacturing run increases the utilization of the single-use materials. Specific labor cost could also be reduced in case of 1) high volumetric productivity at moderately large scale, 2) higher automated process controls and 3) minimal turnaround work particularly with the implementation of single-use technologies and fewer turnaround activities (on a yearly basis) due to longer production duration. Price of media is a key factor that affects the production costs as media consumption will be somewhat higher compared to a fed-batch process, but the aforementioned cost reductions/savings can negate this potential disadvantage. For continuous downstream purification (and here we’re using a continuous product capture step as an example), the one-time cost of resin is reduced dramatically. The buffer usage may be reduced as well. Even though the initial downstream equipment costs are higher to implement continuous downstream processes, overall, the total costs for this step on a product by product basis are reduced through a continuous processing approach.
Question 9
What is the typical time from target to manufacturing?
As I understand the question, you are inquiring about the timeline of a novel lead therapeutic from preclinical development to initiation of the first clinical manufacturing run. If a good platform process development strategy has been established, the overall timeline won’t be significantly different from that of a fed-batch process. Specifically, in cell line development and clone selection there will be additional studies on cell line stability and robustness compared to a fed-batch program but this additional work can be conducted in parallel with other development activities thus not impacting the overall development timeline to a great extent. During cell culture process development, the duration of each run will be longer than fed-batch but the total rounds of studies and number of bioreactors can be greatly reduced given that the performance of the host cell line and media has been previously well-characterized as part of the platform development process. Therefore, the timeline of continuous process development will be comparable or perhaps slightly longer than that of a fed-batch program. Finally, once the process has been locked, the process can be directly scaled up from benchtop models to tox or clinical scale at 50 L or 200 L in most cases (or 1000 L if needed), thanks to the high batch productivity and good process robustness typical of perfusion cell culture. We’ve routinely accomplished preclinical development to GMP production on multiple projects in 18-24 months. Programs that fit well with our platform process fall into the 18-20 month range.