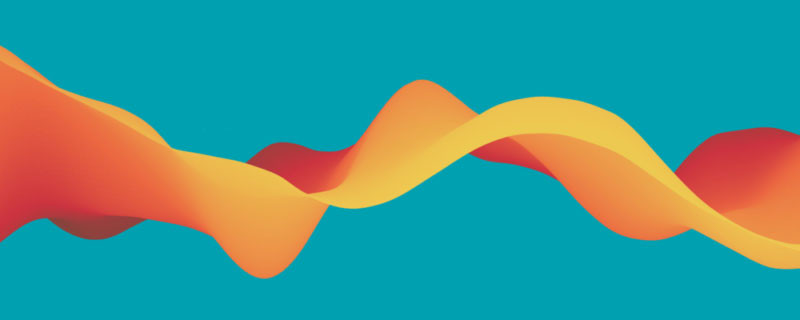
Microfluidic Transfection Device is Poised to Remove Current Transfection Limitations for Gene Therapy Applications
The transfer of materials into cells via transfection is a critical operation of manipulation and engineering of cells. DNA is often used as the cargo for transfer; however, other cargos may include RNA, proteins, small molecules, and ribonucleoproteins, such as those used in the engineering of cell therapy products1,2.
Even though cell transfection was initially developed decades ago, challenges remain with the process. Current methods may damage cells, require special reagents, that may be mixing and time dependent, and often inefficient. Performance may suffer since many approaches rely on the passive diffusion of material into cells, usually though temporarily, induced holes in the cell membrane. These challenges, in turn, create burdens in many areas of current biomanufacturing such as manufacturing at scale 3,4 or in the development of complex, multiple gene-edited cell-based therapeutics 4-6.
Emergence of a robust solution
CellFE has recently announced the development of a microfluidic platform that has the potential to remove many of the current bottlenecks in transfection-dependent production processes. The current and potential capabilities of the device are impressive. The platform can enable the efficient transfer of cargo that is “payload agnostic” as far as type, size, and multiplicity of payload 7-10. It functions in a format that is easily scalable to process the 100’s of liters of cultured cells. This novel device by CellFE is currently available for evaluation and validation by leaders in industry and academia.
Microfluidic design-the key to gentle, efficient, and large-scale transfection
Examination of the design (Figure 1) shows the potential of this device to become a game-changer for the industry. The basic unit of the device is a unidirectional flow cell. The upper surface of the channel contains a series of chevron-shaped ridges. Cells flow though the channel at a precise rate and are rapidly compressed as they pass under each ridge.
In the space following each ridge, the cell recovers to its equilibrium state before encountering the next ridge, which in turn, leads to another compression-equilibration cycle. With each recovery phase the cells take up a small amount of cargo from the surrounding medium. Thus, the cells experience a series of exceedingly rapid compression and recovery cycles suited to the robustness of the cells. The net result is that cells enter the flow channel and emerge as transfected, viable cells that have undergone multiple cycles of cargo import.
This unique mechanism generates an import process that is active and convective rather than being passive and limited by time-dependent diffusion. The convective process is analogous to liquid being drawn into a sponge following compression and release.
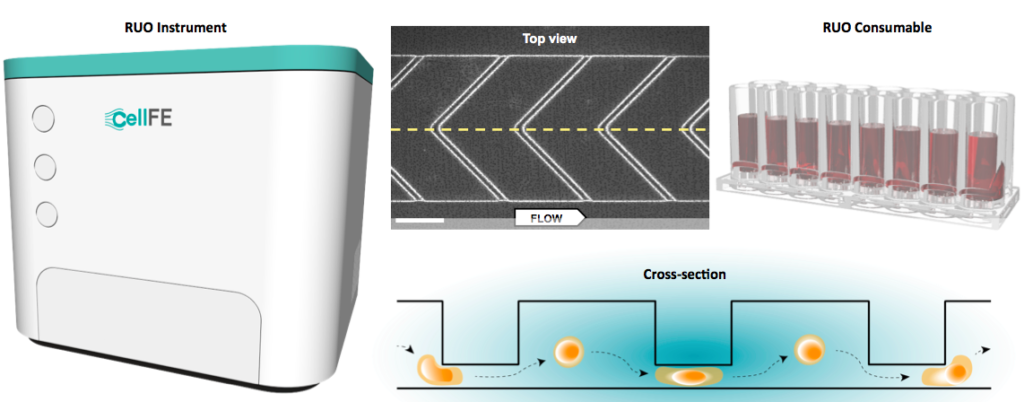
The active, convective import process leads to great efficiency. Efficiencies in the range of 80-90% are typically observed. Studies to date show that efficiency is similar to, or greater than current transfection processes. This high efficiency relative to many existing processes can result, in turn, to less plasmid usage and lower costs.
Enabling for a variety of cell types
The CellFE device was designed to be gentle on the most sensitive cells such as primary T-cells9 and stem cells10, that often suffer from low product yield or low cell recovery. Additionally, the device can be used across a broad range of cell types, making it superior to other technologies currently on the market. Lastly, the device is not limited to cells grown in suspension culture as adherent cells that have been released from their support can be processed as well.
The device delivers healthy functional cells with no observable stress. There is no observed impact on proliferation, immunophenotype, or exhaustion of cells after processing. Cell viability is typically maintained at high levels (90%). Cells can proliferate, activate, and have the same phenotype as when they entered.
Simple to operate and highly scalable
CellFE’s device is simple to deploy and easy to operate. The cargo is placed into the cell culture media prior to entering the device. There are no reagents, incubation periods, or intricate mixing and timing processes.
The system is highly efficient, with a robust flow capacity in the range of 10 million cells/min for each channel. Scale up is easily achieved by simply adding more channels until the desired scale is reached. (Figure 2). For example, CellFe’s clinical scale device is 10 channels and will process 100 M cells/min. For larger bioprocessing applications of 100 or more channels, the device is configured to process in excess of 1 B+ cells/min. The platform’s elegant design and flexibility enables 100’s of liters of cells to be processed in a single pass.
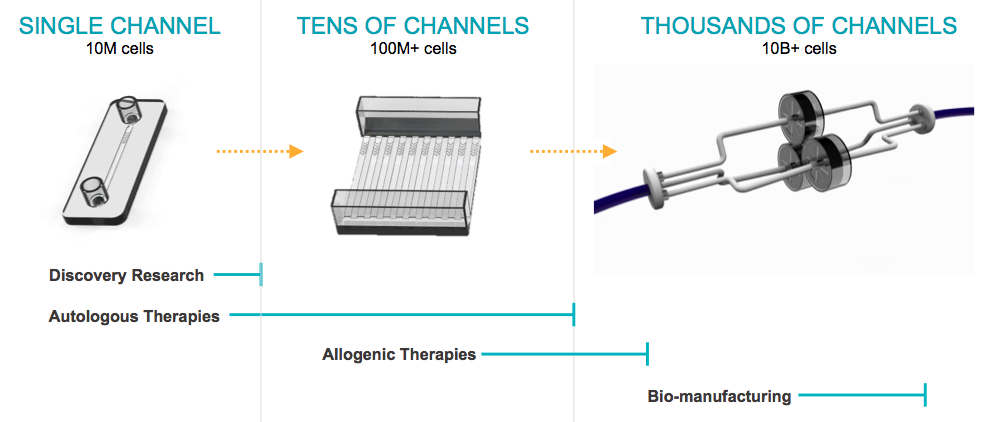
Another key advantage to the CellFE platform is that there is little to no additional development time required to achieve production scale capacity. Since the cells experience the same environment whether there is a single channel or 100’s of channels, once the upfront development is complete, scale-up is straight forward. The time and cost savings to industry partners is extremely significant because with other transfections technologies this iterative process can take weeks or months to optimize and scale3. With the CellFE platform, optimization is completed upfront and can scale directly from R&D to bioproduction. There is currently no product on the market that allows direct scaling from bench to clinic and represents a substantial market opportunity.
Payload “agnostic”
Another key advantage of CellFe’s platform is being payload agnostic. Multiple cargos can be transferred concurrently and the convective process readily enables the transport of larger molecules such as plasmid DNA (>10 Kb), mRNA, RNP, or large proteins with similar efficiency as smaller molecules.
An in-line, closed-loop, transfection device
CellFE’s unique platform is ideally deployed as part of an in-line closed loop system. For example for cell manufacturing, the device could be configured to sit between two bioreactors enabling the transport of cargo into cells at a bioreactor scale. In the cell therapy space, in-line functionally enables the next generation of point-of-care therapeutics.
A generational improvement over previous microfluidic transfer devices
First generation microfluidic devices showed great promise, however they fell short in some of the key areas.
One limitation is that they lost efficiency when processing large cargo, such as plasmids. Another limitation was their inability to scale efficiently. The larger cell volumes needed for clinical and bioproduction applications were not consistently achieved.
In contrast, as a next generation microfludic platform, CellFE’s technology was designed to address many of the limitations of the first generation devices. It not only efficiently delivers large cargo, such as those required for gene-engineering or viral vector production, but it easily scales enabling processing at clinic and bioproduction levels. In short, CellFe’s next generation microfluidic platform is poised to help usher in a golden age in precision gene engineering and bioproduction.
CellFe is amenable to the development improvements or new features in the device. For example, it may be possible to design as recirculation loop to enable the reuse of plasmid, and thus significantly reduce the usage of expensive GMP-grade plasmid.
Benefits Industries with Current Challenges
CellFe’s technology has the potential to disrupt a number of industries, however, in the near term they are focused on three specific markets which are experiencing manufacturing constraints due to transfection technology limitations.
Autologous cell therapy: Currently, there are three main challenges with developing these therapies. First, there are limitations due to the personalized nature of the manufacturing process. Second, because these therapies are used in late-stage cancer patients, they need to be manufactured quickly. Finally, these therapies require complex cell gene editing which are tricky to manufacture. CellFE’s platform is well suited to solve these three challenges. First, its technology results in higher yields of functional cells. Second, it allows for the delivery of large, difficult, and multiple payloads. Lastly, the in-line functionality of the device should facilitate rapid manufacturing.
Allogenic cell therapy: Currently these therapies suffer from poor manufacturing scale-up efficiency and the need for them to perform multiple cellular gene edits. CellFE’s technology solves these challenges by producing a higher yield of functional cells and the ability to scale to 100’s of liters of cells.
Viral vector production for gene therapy: Currently, the majority of gene therapy vectors are generated by polyethylenimine (PEI) transfection of multiple, often large plasmids into bioproduction cell lines such as HEK 293T2. As a result, the efficiency of transfection can suffer. Moreover, current PEI transfection methods are limited at scale to a few dozens of liters per batch3. This presents a huge constraint when large bioreactor-size production is needed for a therapeutic application. Other transfection challenges include the long development time for the optimization of PEI transfection at larger scale-which can extend from weeks to months3. CellFE’s device could help solve these challenges because it is scalable to bioreactor volumes, can function in a closed-loop, and should reduce the use of reagent and DNA. Further, the use of CellFe’s device will simplify the manufacturing process and dramatically increase product throughput. If the increased production efficiency is achieved, it would, in turn, significantly reduce the cost of many therapies.
In summary, CellFE has developed promising next-generation microfluidic transfection technology to enable lower-cost, high yield therapies at scale. This emerging technology has the potential to disrupt numerous industries that utilize transfection in their production processes.
To learn more, please see CellFE Microfluidic Technology
Footnotes
-
1. Doudna JA, Charpentier E. The new frontier of genome engineering with CRISPR-Cas9. Science. 2014;346(6213):1258096. doi: 10.1126/science.1258096
-
2. Sadelain, M., Rivière, I. & Riddell, S. Therapeutic T cell engineering. Nature 545, 423–431 (2017).
-
3. Insights on Successful Gene Therapy Manufacturing and Commercialization (Ebook). Accessed Jan 10, 2021:
-
4. Bolhassani A, Khavari A, Orafa Z (2014) Electroporation–Advantages and drawbacks for delivery of drug, gene and vaccine. Application of Nanotechnology in Drug Delivery, ed Sezer AD (InTechOpen, London).
-
5. Cox DBT, Platt RJ, Zhang F (2015) Therapeutic genome editing: Prospects and challenges. Nat Med 21:121–131.
-
6. Yin H, et al. (2014) Non-viral vectors for gene-based therapy. Nat Rev Genet 15: 541–555.
-
7. Liu A et.al. (2018) Microfluidic generation of transient cell volume exchange for convectively driven intracellular delivery of large macromolecules. Mater Today (Kidlington);21(7):703-1
-
8. H.Nejadnik et. al., (2020)Instant labeling of therapeutic cells for multimodality imaging. Theranostics. 10(13): 6024–6034
-
9. Sicher, I. et al. Novel Microfluidic Platform for Scalable Transfection of CRISPR/Cas9 RNP in Human T cells (Poster). Accessed April 21, 2021:
-
10. Han S. et. al. Microfluidic Device for Intracellular Delivery of Nucleic Acids Into Human CD34+Hematopoietic Stem and Progenitor Cells, ASGCT 23rd annual meeting, May 12-15, 2020