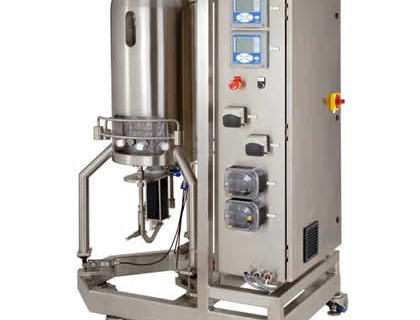
Single-use Perfusion Culture Enables Continuous Bioproduction
Guest blog by William G. Whitford
Sr. Manager, HyClone Cell Culture, GE Healthcare, Logan, UT
Glossary
- Single-Use Systems: Bioprocessing equipment containing (usually plastic) components that are used in one manufacturing cycle and then permanently retired.
- Mechanically Agitated Suspension Bioreactor: Bioreactors supporting the culture of suspension or matrix-bound cells (eg, microcarriers) through continual mixing (eg, by an impeller).
- Continuous Bioproduction: Production of biological products using continuous end-to-end processes and an integrated control strategy– from starting materials to final dosage units. Also can refer to unit parts of the process operated in a continuous flow.
- Perfusion Culture: The culture of cells by way of their isolation followed by the exchange/renewal of either the culture medium metabolites and gasses or the whole culture medium fluid itself.
- Single-use Perfusion Bioreactor: Single-use bioreactors supporting some type of perfusion culture. In bioproduction it’s implied that they are of a manufacturing scale and quality.
- Filter Bioreactor: Supports perfusion culture through the retention of matrix (eg, microcarrier)-bound or suspension cells by an external or internal (eg, fixed, floating or spinning) porous membrane or screen.
- Packed bed Bioreactor: Supports culture of either suspension or adherent cells within an integral macro-porous matrix.
- Hollow Fiber Perfusion Bioreactor: Supports culture of suspension or adherent cells employing an array of integrated micro-porous hollow fibers.
- Centrifugation-based Perfusion: Supports perfusion culture without such invasive components as membranes, through the retention of matrix (eg, microcarrier)-bound or suspension cells by continuous flow centrifugation of various rotor design.
- Acoustic Wave-based Perfusion: Supports perfusion culture through the retention of cells/microcarriers by ultrasound-based isolation.
Single-Use Technology
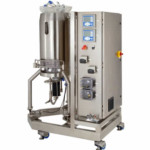
Many valuable features are provided by single-use (SU) technology, including lower initial investment, facility and operating costs. Biomanufacturers now employ significant SU technology in the majority of their cell culture applications. Most upstream operations are now supported by SU, including media and process liquid preparation and cell culture for seed generation and production. Single use bioreactors are now available up to a 2000L working volume (1). A number of diverse sparging (mass-transfer) and cell suspension designs are available. These include various packed bed reactors and rocker-style, top or bottom-mounted impeller and orbitially shaken mechanical agitation devices (2). As flexible bioprocessing modules and plug-and-play factories gain acceptance, we can expect facilities with higher-level, integrated system management (3).
Continuous Processing
Many valuable features can be provided by continuous processing (CP) such as increased product quality and reductions in plant construction/operating costs (4). In CP, materials continuously flow between pieces of equipment as they are continually processed into intermediate or final products. They operate in this way for various lengths of time — from days to months. CP replaces the discontinuous “batch” production mode, where a defined quantity of product is produced in a unique, limited volume and defines one cycle of manufacture. Batch production is usually segmented into many individual steps —and even performed at separate suites, buildings or sites. Batch processing often involves significant intermediate hold and transport processes. CP, on the other hand, occurs without interruption at a single location. A limited portion of the manufacturing train can be referred to as “continuous”, and it doesn’t have to refer to the entire process as a whole.
Continuous Bioproduction
Many benefits are provided by continuous processing as applied to biomanufacturing, or continuous bioproduction (CB). They include reductions in classified suite extent, personnel requirements and operational steps. Applications of CP techniques in bioproduction have become more popular in the past few years and some are even predicting even an eventual dominance in the industry. Many modern upstream bioproduction approaches already use underlying technologies that can support simple conversion to CB. It is encouraged by regulatory agencies and provides a number of tangible benefits. Surprising to some, continuous processes are being applied by a growing number of biopharmaceutical manufacturers in many unit operations (5). Upstream, a variety of manufacturing-scale perfusion-ready or perfusion-capable bioreactors are currently in use or are becoming available. Successful perfusion culture even exists in GMP installations, including for approved product. Genzyme’s new Geel, Belgium plant houses 4000L perfusion bioreactors.
CB promotes operational excellence (OpEx) tools and goals supporting PAT and QbD initiatives. It easily incorporates new in/on-line monitoring and real-time quality assurance techniques. Our increasing process understanding supports CB, and CB values in turn advance continuous quality verification (CQV), continuous process verification (CPV), and real-time release testing (RTRT) enabling initiatives. Both these as well as heightened process control are promoted by the appearance systems for the SU, automated withdrawal and processing of whole or even cell and microcarrier-free samples. These samples can be automatically routed for online, multi-analyte or multivariate monitoring.
Questions have been raised regarding CBs performance reliability, validation complexity, integrated control requirement, economic justification and lot definition. Challenges do exist in such areas as 1) validation of equipment (including SU) in extended operation, 2) mass transfer at the high cell densities of experienced, 3) end-to-end CB process control and 4) surface fouling and cell-line stability in extended operations.
SU Continuous Bioproduction
Advantages to the combination of these novel approaches to biomanufactring are many and include that it heightens process flexibility, efficiency and capability, as well as product consistency (6). CB supports a continuously variable manufacturing rate and promotes “numbering-up” of production units to increase capacity. SU CB supplies a comprehensive process flexibility due to its equipment being so easy to clean, inspect and maintain. It supports ease of product changeover because it is very modular, reconfigurable and transportable.
CB supports a reduced process development time and this complements such SU efficiencies as its elimination of any cleaning and steaming service development time. SU flexibility is very complementary here and includes open architecture and “hybrid” implementations. Examples of this include combinations of reusable and disposable systems as well as employing multiple suppliers, locations and particular equipment styles. We must also consider the newer SU transfer tubing/systems, connection technologies, distribution manifolds, container porting and fluid impulsion becoming available. These are of great value when designing processes with entirely new flow paths, process monitoring and control, or lot designations – such as in the case of CB.
A continuous strategy can shorten the process stream and manufacturing duration, reduces downtime and nearly eliminates the (manual) handling of process intermediates. As CB processes have greatly simplified production trains they inherently facilitate application of closed processing approaches. The modularity and integral gamma irradiation sterility of SU combined with the simplicity and sustained operation of CB promise the appearance of platforms with lower processing times and heightened operational ease. SU equipment in continuous bioprocessing promotes the design of closed and highly integrated operations. This is enabling such approaches as the growing “Factory-of-the Future” initiative of manufacturing even different product types in single greyspace (or ballroom) suits of reduced classification.
Controlled non-classified (CNC) is a term referring to noncritical areas in GMP manufacturing facilities. Bioproduction processes employing closed operations in an area of reduced classification are being actively pursued. The integral nature of such connected (contiguous) operations as continuous processes present lend themselves to a (functionally) closed operation. By exploiting these system features a facility can be envisioned with combined work areas not requiring classification. Such a facility offers such benefits as a reduction in 1) facility construction utilities and costs 2) manufacturing suite area and classification, 3) maintenance of the above, and 4) manufacturing operation steps and costs.
Recent trends in manufacturing demands also support the above goals. Examples of this include personalized medicines, lower mass “next-gen” products, globalization, regionalized production demands, price-sensitive and controlled markets, growth of multi-product facilities and the demand for localized pandemic response.
CB batch is primarily determined by run time rather than bioreactor volume, thereby reducing the size of a bioreactor required. This supports SU limited reactor size availability . Also, reducing such non–value-added steps as intermediate product hold and final product inventory reduces the faculty extent and QS requirements for their storage. This complements SU technology and systems that inherently present reduced controlled environment extent, validation requirements, quality systems maintenance, and operations personnel.
In fact, there really are a few real financial, engineering or regulatory concerns in the consideration of SU CB for pharmaceutical manufacturing. Many envision closed, disposable, integrated and continuous bioproduction systems for biopharma in the near future,
Perfusion Culture
Perfusion culture is virtually the only CB-supporting upstream process available. Operation-specific features provided by perfusion include significantly reduced product-reactor residency duration and a dramatic increase in reactor-volumetric productivity. In the perfusion mode, cells are retained or immobilized in some way to allow used culture medium to be replaced by fresh medium. Modern serum-free, defined media formulations support production in very high perfusion culture cell densities. Remarkably, peak densities (albeit transient) of 200 x 106 cells/mL have been achieved.
Process equipment supporting perfusion include many flavors of fluidized and packed bed, centrifugal concentrators, gravity based (conical and inclined ramp) settlers, hydrocyclones, spin-filters, ultrasonic resonators/filters, as well as crossflow membrane and diverse (internal and external) hollow fiber based systems. Perfusion culture-based processes now support a variety of protein biologicals, vaccine and cell therapies. Here we look at manufacturing scale bioreactors engineered with SU components.
Perfusion culture can place increased pressures upon manufacturing systems. This includes validation of operation throughout the duration of a significantly extended culture. While some perfusion technologies and materials could support indefinite operation, most currently consider culture durations of between 20 and 60 days.
Green initiatives and sustainability goals are supported as CB reduces the equipment to be CIP/SIP as well as the services and energy required for these operations. Environmental objectives are supported by the fact that CB operations can reduce the amount of equipment to be cleaned or steam sterilized, process steps, service requirements and therefore energy consumption. Reviews and environmental impact assessments for CB manufacturing approaches and SU materials come out remarkably favorable to the approach (7).
Single-use Perfusion Bioreactors
There have been research SU hollow fiber perfusion bioreactors available for over 40 years. However, only recently have commercially available SU production-scale perfusion-capable equipment become available.
SU and hybrid production-scale perfusion equipment has appeared in support of the majority of process formats, platforms and modes. SU perfusion equipment is currently available supporting mechanically agitated suspension, hollow fiber, floating filter and packed bed reactors. When fitted with appropriate ancillary equipment most large-scale SU bioreactors are capable of providing perfusion culture. Some, such as packed-bed reactors, inherently lend themselves to perfusion operation. The bioreactor accessories commercially available to support SU perfusion culture include hollow fiber exchange, continuous flow centrifugation and acoustic wave separation.
Mechanically Agitated Suspension Reactors
Mechanically-agitated (stirred-tank) bioreactors, containing either suspension or anchorage-dependent cultures on a support matrix, have dominated the bioproduction industry. The stirred tank bioreactor (STR) is the most popular of the suspension systems for such reasons as it is simple to operate, easy to scale up, and well understood. For attached cultures, several types of commercially available microcarriers include dextran-based, glass and plastic microcarriers. STRs are easily converted to continuous processes through the addition of ancillary perfusion enabling devices (see SU Accessories Supporting Perfusion Culture below).
Fixed/Floating Filter Bioreactors
Pre-sterilized SU wave-action (or rocking) bioreactors provide another perfusion-capable solution. In this case disposable bags with no integral impeller are rocked to provide oxygen transfer. Some are available with integral perfusion culture capability and employ an innovative floating filter that is kept clean and unclogged by the wave motion. This establishes a simple, disposable perfusion bioreactor ideal for various biotechnology processes. Many can also support an external cell immurement based perfusion.
Packed bed Bioreactors
Packed bed (PB) bioreactors are a type of entrapment culture that can maintain a variety of cell types for long periods of time. PB reactors providing such features as extremely low shear due to their macro-porous matrix immobilization of cells and are currently employed in a number of culture applications. They macro-porous (PET) fibers present a large number of inter-connected, open pores. Mass transfer, and therefore kLa, can be quite different from those for typical stirred tank reactors. kLa values can also differ greatly between the various packed bed reactor styles, based upon such factors as differences in relative working volumes and recirculation rates.
Hollow Fiber Perfusion Bioreactors
Hollow Fiber Perfusion Bioreactors (HFPB) are yet another type of high-density immurement culture. While not a stirred tank suspension-mode type, they aren’t truly packed-bed reactors either. Cells are typically seeded within the cartridge body, but outside of the hollow fibers in the “extracapillary space” (ECS). In this configuration, culture medium is pumped through the hollow fibers allowing both products and nutrients to diffuse through the fiber walls in each direction. Culture medium from the cartridge can be oxygenated within this the loop, or it can be ported out for collection as fresh medium is introduced. The basic features of a HFPB system include a very high culture binding surface-to-volume ratio, immobilization of cells at biomimetic (very high) density and a selectable porosity in the fibers .
SU Accessories Supporting Perfusion Culture
Hollow Fiber Media Exchange
A number of external hollow fiber-based perfusion-supporting devices exist, and most providing manufacturing-scale operation are now available in SU. Benefits include nearly linear scale-up, simplicity of operation and validation, and choice of filter materials and pore size. The operating parameters in a hollow fiber perfusion process have to be optimized to prevent filter clogging in time by cell aggregates in the pores or accretion of debris across the membrane surface. Maintaining a high viability in the culture, optimizing pump speed and measuring filter membrane pressure are three key aspects to consider for a successful hollow fiber perfusion culture.
Continuous Flow Centrifugation
A wide variety of rotor designs are available for centrifuges presenting a high capacity continuous flow− with some enabling a perfusion mode of cell culture. Each is economical to use and even the single use components can withstand the forces throughout perfusion runs. They can provide consistent performance and establish processes that are easy to transfer and scale-up. However the absence of filters means that some cells will pass through to the permeate stream.
Upon installing the centrifuge main module and tubings using an aseptic technique the circuit becomes, for practical purposes, a closed system. Different products are based upon unique separation principals, but in all the cell suspension is fed into one end of the module and centrifugal force supports separation of the media from the increasingly concentrated cells. This provides low-shear isolation of cells with minimal reduction in viability.
Acoustic Wave Separation
Separation by ultrasound provides for the isolation of small particles from fluids without using invasive materials or moving components demanded in spin filters or centrifugal approaches. These cell retention devices are often referred to as ultrasonic separators, filters or resonators or as acoustic wave separation (AWS). The basic principal has been in application for many years, but has had practical success only recently in large-scale animal cell separation for perfusion culture or reactor harvest applications.
Ultrasonic separators have a chamber where the original signal is converted into a standing acoustical wave field that severely limits dispersion of the cells flowing through the chamber. The acoustic energy establishes a “virtual” screen or mesh providing a low-shear, non-contact, non-fouling, non-moving means of cell separation.
Spinfilters
Both internal and external spinfilters of many designs have been successfully used for decades. They develop a tangential flow of medium across a screen to prevent it’s clogging with cells during perfusion culture. The filtering mechanism is generally made from layers of stainless steel mesh. Some suppliers now provide SU units of varying sizes for use with many cell culture platforms. The main body of the disposable unit is polycarbonate and features a filtering open mesh of highly specialized monofilament fabric.
Conclusion
Continuous processing is a very successful approach in much modern manufacturing. Perfusion culture provides such benefits in bioproduction as improved product consistency and supports such CP benefits as simplified production trains and reduced handling of intermediates. The operational efficiencies and flexibility of SU systems complement these features. Recent bioproduction trends, such as modular architectures, synergize with both single-use and CP benefits. We are seeing growing interest in SU perfusion bioreactor-supported continuous bioproduction inititives.
For more information on Continuous Bioproduction, please see “Continuous Processing: From Cookie Preparation to Cell-based Production.“
References
- Eibl, R., Kaiser, S., Lombriser, R., Eibl, D. (2010) Disposable bioreactors: the current state-of-the-art and recommended applications in biotechnology. Appl Microbiol Biotechnol. 86(1):41-9.
- Galliher, P and Pralong, A. (2013) When the Process Becomes the Product: Single-Use Technology and the Next Biomanufacturing Paradigm BioPharm International Supplements, Volume 26, Issue 4, pp. s27-s30.
- Levine, H.L., Lilja, J.E., Stock, R., Hummel, H., Jones, S.D. (2012) Efficient, flexible facilities for the 21st century BioProcess Int. 10 (S11) 20–30.
- Schaber, S.D., Gerogiorgis D.I., Ramachandran R., Evans J.M., Barton P.I., Trout B.L. (2011) Economic analysis of integrated continuous and batch pharmaceutical manufacturing: a case study. J. Ind. Engin. Chem. 50 (17), 10083–10092.
- Warikoo, V., Godawat, R., Brower, K., et al. (2012) Integrated continuous production of recombinant therapeutic proteins. Biotechnol. Bioeng. 109(12), 3018–3029.
- Whitford, W.G. (2013) Supporting continuous processing with advanced single-use technologies. BioProcess Intl. 11(4) (Suppl.) 46–52.
- An Environmental Life Cycle Assessment Comparison of Single-Use and Conventional Bioprocessing Technology. Source: GE Healthcare Life Sciences http://www.bioresearchonline.com/doc/an-environmental-life-cycle-comparison-conventional-bioprocessing-0001.
Author Particulars
William Whitford, Sr. Manager, HyClone Cell Culture, GE Healthcare, Logan, UT
Bill Whitford is Sr. Manager, HyClone Cell Culture, GE Healthcare in Logan, UT with over 20 years experience in biotechnology product and process development. He joined the company nine years ago as a team leader in R&D developing products supporting biomass expansion, protein expression, and virus secretion in mammalian and invertebrate cell lines. Products he has commercialized include defined and animal product-free hybridoma media, fed-batch supplements, and aqueous lipid dispersions. An invited lecturer at international conferences, Bill has published over 250 articles, book chapters and patents in a number of fields in the bioproduction arena. He now enjoys such biomanufacturing industry activities as serving on the editorial advisory board for BioProcess International.