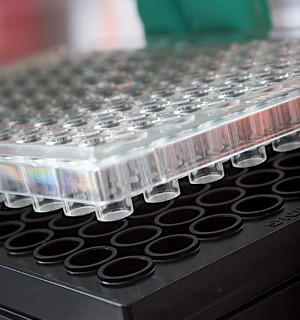
A Novel 3D Immune Oncology Model for High Throughput Testing of Tumoricidal Capability
Introduction
Immunotherapy, particularly for cancer treatment, is a very active area of both academic and clinical research. There has been increased interest in utilizing a patient’s own T cells to attack their tumors. However, positive laboratory results do not always translate from bench to the clinic, and the effectiveness of immune cells therapies are not equivalent for all patients or cancer types. To better understand these differences, there is a need for improved in vitro oncological models that more closely resemble the tumor environment in vivo. Traditionally, tumoricidal activity and immune evasion have been studied by utilizing two dimensional systems (2D) which may not accurately reflect the complexity of a three dimensional (3D) tumor. The physical barriers immune cells need to overcome in a 3D system are much greater than those of 2D. Not only do immune cells need to migrate to the tumor site, but they also need to infiltrate a 3D structure in order to attack the target cells. Furthermore, it has been shown that phenotypic differences occur in 3D-cultured tumor cells that allow for a higher resistance to cytotoxicity1-2. Here, we demonstrate a novel, high-throughput 3D model to study cancer/immune cell interactions by combining two commercially available products; 96 well ultra-low attachment microplates and 96 well permeable support systems. By replacing the standard 2D flat-bottom permeable support receiver plate with an ultra-low attachment microplate, an easy-to-use, 3D, high-throughput assay to investigate immune cell homing, tumor cytotoxicity, and tumor immune evasion is achieved.
Materials and Methods
Immune Cell Migration. NK-92MI (ATCC® Cat. No. CRL-2408) cells were stained by incubation with 80 µM CellTracker™ Blue CMHC Dye (Molecular Probes™ Cat. No. C2110) for 1 hour. After labeling, 150,000 cells were added to each insert of a Corning® HTS Transwell®-96 Tissue Culture System (Corning Cat. No. 3387) and allowed to migrate overnight towards various concentrations of Human stromal-cell derived factor-1 (SDF-1α) / CXCL12 (Shenandoah Biotechnology Inc™ Cat. No. 100-20) in Iscove’s Modification of DMEM (IMDM, Corning Cat. No. 10-016-CM) supplemented with 10% fetal bovine serum (FBS, Corning Cat. No. 35-010-CV). Migration was quantified by measuring Cell Tracker™ Blue fluorescence via flow cytometry using the Miltenyi Biotec MacsQuant® and expressed as a ratio of cells in the basolateral chamber compared to total cells seeded in the insert.
Tumor Spheroid Formation and Immune Infiltration. A549/GFP Cells (Cell Biolabs, Inc. Cat. No. AKR-209) were seeded into 96 well spheroid microplates (Corning Cat. No. 4515) at 2,000 cells per well in 100 µL of IMDM supplemented with 10% FBS. The plate was incubated 24 hours to enable spheroid formation.
After spheroid formation, 100 µL of medium or varying concentrations of effector cells were added to A549 spheroids for overnight incubation. Specifically, we used both NK-92MI and MOLT-4 (ATCC Cat. No. CRL-1582) cells pre-labeled with CellTracker™ Blue CMHC Dye. Morphology of the spheroid with NK-92MI infiltration was examined by immunohistochemical staining of CD45 and e-cadherin. The impact of effector cell type and number on the spheroids was assessed by analyzing the dissociated spheroid. Briefly, culture medium was removed and replaced with 150 µL TrypLE™ Select Enzyme (10X) (Gibco™ Cat. No. A1217701). Spheroids were incubated at 37°C until they could be broken up into single cells with minimal pipetting. Fluorescence of the dissociated cells was quantified via flow cytometry. A549 percent viability was calculated relative to A549 cell count in the absence of effector cells.
3D Immune Oncology Model. 2,000 A549/GFP cells per well were seeded into 96 well spheroid microplates. The next day, medium was replaced with 200 µL of IMDM 10% FBS containing 30 ng/mL of SDF or vehicle control. NK92-MI cells were stained, as previously described, while simultaneously being treated with 2 µg/mL prostaglandin E2 (PGE2) (Tocris Cat. No. 2296) or vehicle control in serum-free IMDM for 1 hour. HTS Transwell-96 well Permeable Supports were placed in 96 well spheroid plates (a schematic is shown in Diagram 1). NK-92MI cells were then re-suspended in serum free IMDM and seeded onto inserts at 50,000 cells/well. After 24 hours, inserts were removed, and spheroid microplates were processed for flow cytometry to calculate percent migration of NK92-MI cells and A549 percent viability.
Results and Discussion
Migration
An important component to any immune response is the activation of immune cells and subsequent relocation to the target area requiring defense. In vitro migration studies allow for evaluating immune cell recruitment to determine conditions which enhance or suppress this activity. In this study, cells from a Natural Killer (NK) cell line derived from peripheral blood, NK-92MI, were placed in the apical compartments of Transwells with 5.0 µm pore size and allowed to migrate overnight towards medium containing various concentrations of SDF-1α, a CXC chemokine that signals through the CXCR4 receptor and known chemoattractant for lymphocytes³. The dose response of NK-92MI chemotaxis (Figure 1) in the present study has an EC50 value of 1.76 nM, which is consistent with values for NK cell lines and primary NK cell chemotaxis reported in literature4.
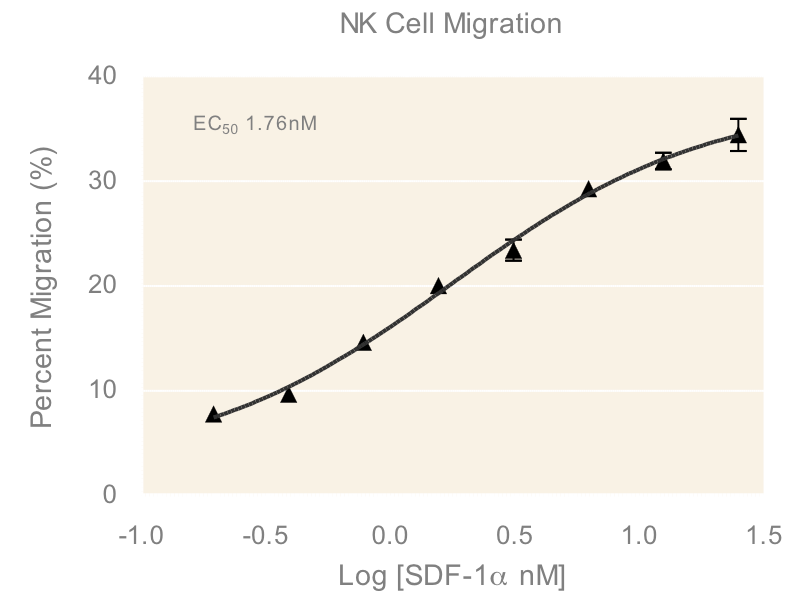
Infiltration
The presence of certain immune cells in a malignant structure has been shown to correlate with increased patient survival5. To this end, 3D models can be utilized to observe immune cell infiltration into the spheroid unlike more commonly used 2D in vitro models for studying immune cytotoxicity. Two common methodologies for visualizing immune cell infiltration are histology and confocal microscopy. Figure 2 shows CD45 (red) and e-cadherin (brown) stained sections of A549/GFP spheroids infiltrated by NK-92MI cells. Infiltration can also be observed via confocal microscopy as shown in Figure 3, with noticeable blue NK-92MI cells interspersed with the green A549/GFP cells of the spheroid. These studies confirm that the immune cells in this model are not only able to reach the target tumor cells, but are also capable of infiltrating the 3D spheroid structure.
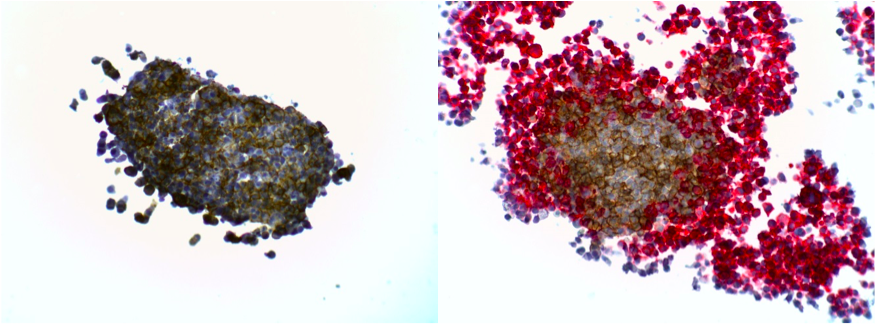
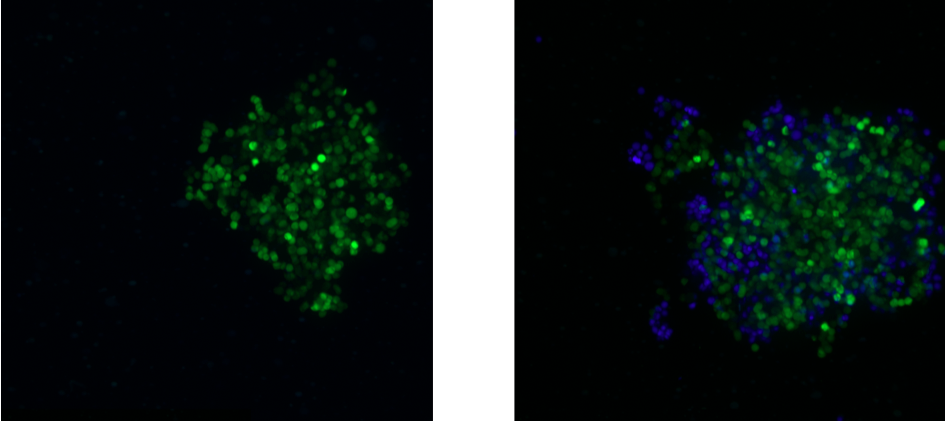
3D Cytotoxicity
To investigate the immune induced cytotoxicity of A549 cells, both NK-92MI and MOLT-4 suspension cells were added to spheroid microplates containing preformed A549 tumor spheroids. NK92-MI cells are known to be cytotoxic to a wide range of malignant cells. The T cell leukemia cell line MOLT-4 were used as a negative control since they have no known cytotoxic effect on other malignant cells. Figure 4 demonstrates the dose dependent effect of NK-92MI cells on A549/GFP viability, in contrast to the limited effect of the MOLT-4 cells which only show cytotoxicity at the highest concentrations. This data supports the specific targeting of the NK-92MI cells to the A549 cells and resulting tumor spheroid cell death.
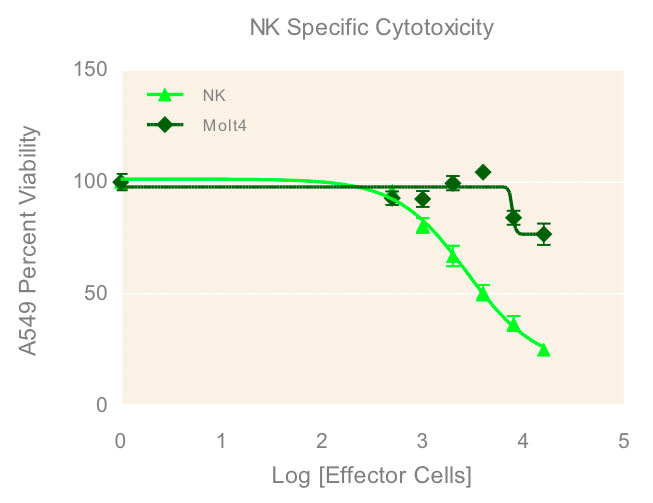
Migration with Cytotoxicity
To evaluate the migration and cytotoxic effects of NK-92MI cells on A549 tumor spheroids in 3D in one single, easy-to-use high-throughput assay, the HTS Transwell®-96 Tissue Culture System and spheroid microplate were combined as previously described (Diagram 1). Figure 5 demonstrates how immune cell migration can be enhanced by the addition of chemokines, such as SDF-1α, as well as suppressed by the addition of inhibitors, such as PGE2. NK migration was significantly increased when the chemoattractant SDF-1α was present in the receiver or spheroid microplate. Conversely, migration was significantly decreased when NK cells were exposed to PGE2, a known inhibitor of immune cell function often secreted by cancer cells as a form of immune evasion6. Tumoricidal activity corresponded well with migration data, with the lowest viability of A549 cells observed with NK cells exposed to SDF without PGE2 (Figure 6).
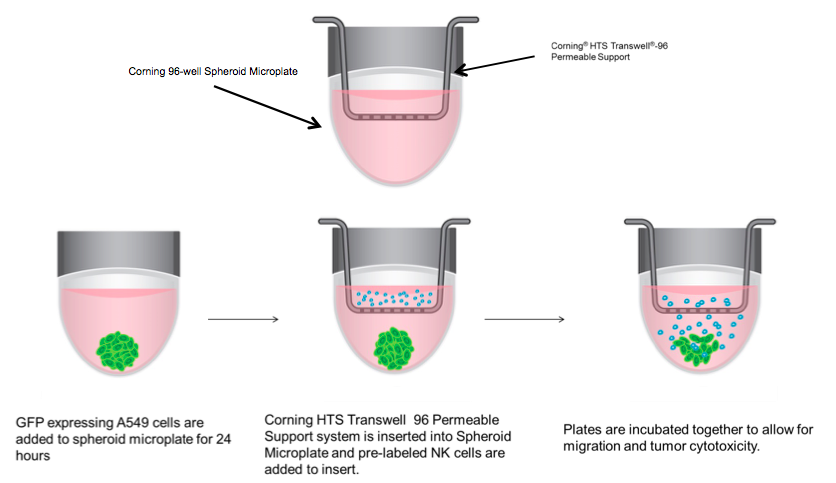
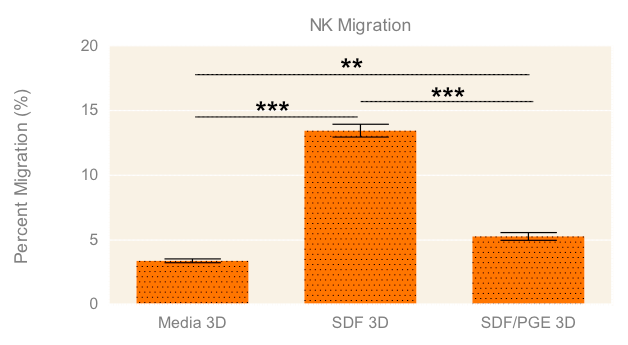
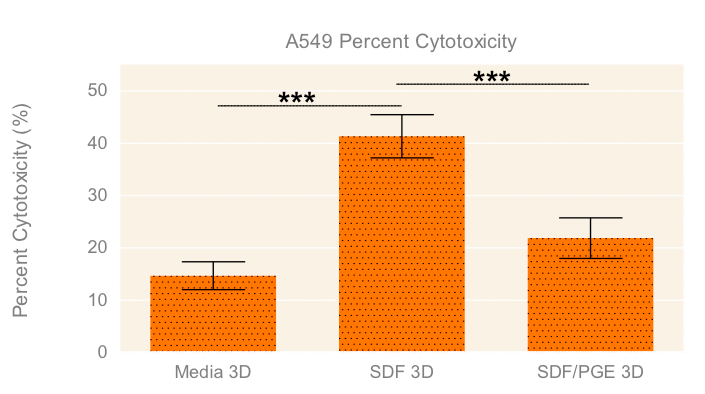
Conclusions
In order to create a model system to investigate immune cell homing, 3D tumor cytotoxicity, and tumor immune evasion in a single, high-throughput assay, we combined a 96 well low attachment microplate and a 96 permeable support system. Previous studies have investigated separate components of this model by looking at either cell migration towards a 2D monolayer of target cells or 3D tumoricidal activity independent of homing and immune evasion. The system described here allows for simultaneous assay of immune cell migration, invasion and subsequent tumoricidal activity resulting in a more comprehensive and in vivo-like immune oncology model.
References
- Holmes TD, El-Sherbiny YM, Davison A, Clough SL, Blair GE, Cook GP., A human NK cell activation/inhibition threshold allows small changes in the target cell surface phenotype to dramatically alter susceptibility to NK cells. The Journal of Immunology, 2011, 186(3), 1538-1545.
- Dangles-Marie V, Richon S, El-Behi M, Echchakir H, Dorothée G, Thiery J, Validire P, Vergnon I, Menez J, Ladjimi M, Chouaib S, Bellet D, Mami-Chouaib F., A three-dimensional tumor cell defect in activating autologous CTLs is associated with inefficient antigen presentation correlated with heat shock protein-70 down-regulation, Cancer Res. 63 (2003) 3682–3687.
- Bleul C C, Fuhlbrigge R C, Casasnovas J M, Aiuti A, Springer T A., A highly efficacious lymphocyte chemoattractant, stromal cell-derived factor 1 (SDF-1), J Exp Med. 1996;184:1101–1110.
- Berahovich RD, Lai NL, Wei Z, Lanier LL, Schall TJ., Evidence for NK Cell Subsets Based on Chemokine Receptor
Expression. The Journal of Immunology, 2006, 177:7833-7840. - Protti MP, De Monte L. Immune infiltrates as predictive markers of survival in pancreatic cancer patients. Frontiers in Physiology. 2013;4:210.
- Holt D, Ma X, Kundu N, Fulton A., Prostaglandin E2 (PGE2) suppresses Natural Killer cell function primarily through the PGE2 receptor EP4. Cancer Immunol Immunother, 2011, 60(11): 1577–1586.