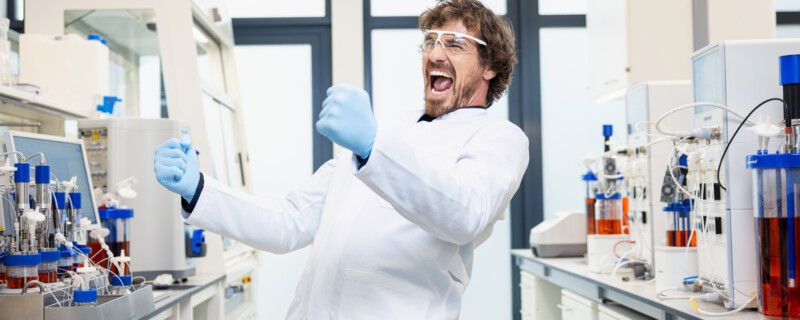
Best Practices for Cell and Gene Therapy Biomanufacturing
Cell and gene therapies (CGTs) are a revolutionary approach in regenerative medicine, offering potential curative treatments for a range of diseases. With the rapid expansion of research and development in this field, there’s a growing focus on allogeneic applications to broaden the reach of these therapies beyond individual patients.
Many CGTs typically begin with primary and stem cells as starting materials. These cells, including T lymphocytes, human pluripotent stem cells (hPSCs), and mesenchymal stem cells (MSCs), are isolated and modified to create innovative therapies. Traditionally, CGTs have relied on 2D static systems, which often face challenges such as lower yields, lack of real-time bioprocess control, and limited scalability to cultivate enough cells for allogeneic use. As such, there is an immense need to develop cell cultivation systems to overcome scalability bottlenecks and facilitate a more straightforward industrialized approach to meet the growing needs of the industry.
To address these limitations, the industry is transitioning to 3D cell culture systems in bioreactor platforms. These systems offer enhanced scalability, real-time monitoring, and process control, enabling more efficient upstream processes. Additionally, leveraging the technologies and expertise of commercial partners can streamline the path to commercialization.
In this discussion, we explore best practices for CGT biomanufacturing, emphasizing the transition to 3D cell culture systems and the importance of collaboration and knowledge sharing in driving successful outcomes and bringing life-changing therapies to market for patients in need.
Implementing Bioreactor Platforms into the CGT Process
Transitioning from static 2D systems to dynamic 3D suspension bioreactor platforms is a key step for improving upstream bioprocesses in CGT development. These advanced bioreactor systems facilitate improved nutrient and oxygen exchange, leading to enhanced productivity and yield for cell-based products, while also enabling scalability for industrial applications.
While not all adherent cell lines can be easily adapted to non-adherent culture, adherent stem cells can be expanded in suspension either as cell-only aggregates or on microcarriers. The size of cell-only aggregates can be controlled through seeding density, stirring speed, and bioreactor impeller design. Microcarriers, typically ranging from 100 – 300 µm in diameter and composed of materials like glass, DEAE-Dextran, polystyrene, or alginate, provide an attachment matrix for adherent cells in suspension culture. Peptides or proteins like fibronectin, collagen, or Matrigel® may be coated on the surface of microcarriers depending on the attachment needs of the cell type of interest. This method has been used successfully to cultivate adherent MSCs in stirred-tank bioreactors. Some general guidelines for microcarrier MSC cultures are provided in Figure 1.
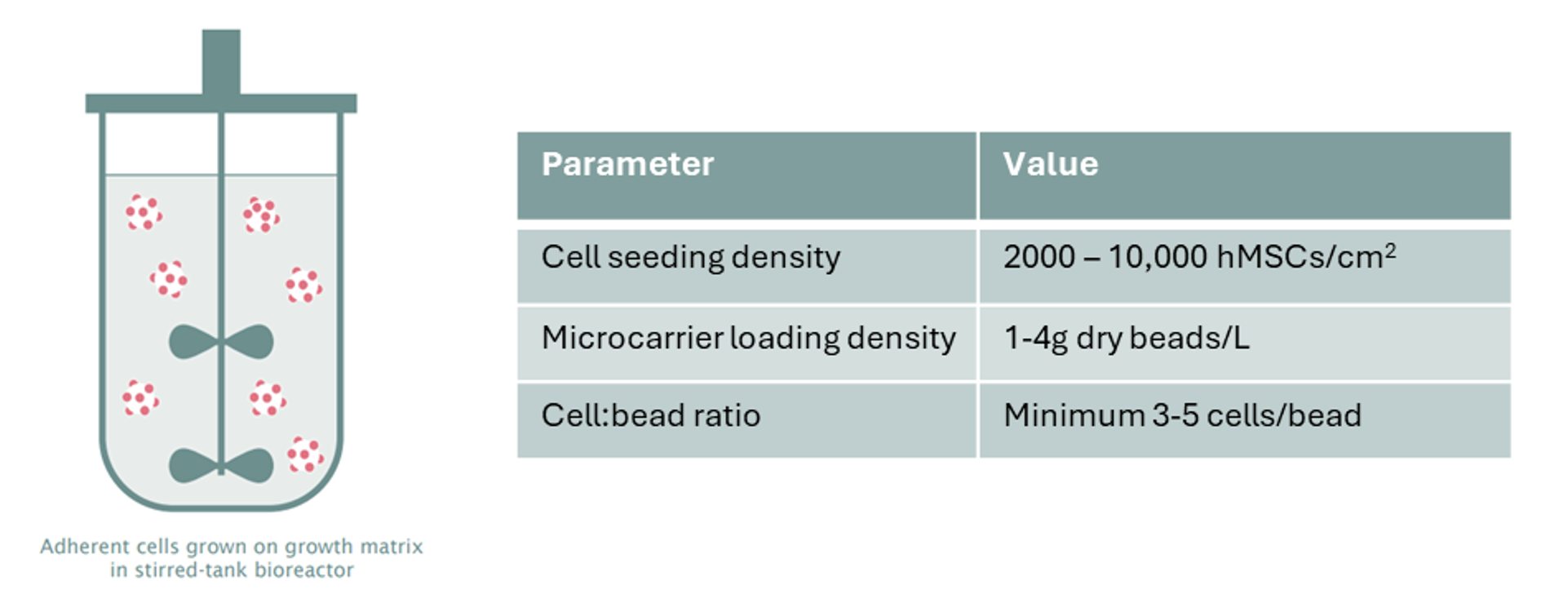
Single-use stirred-tank bioreactors, like the BioBLU® Single-Use Bioreactors from Eppendorf, are ideal for culturing culturing T cells and high-quality stem cells. With working volumes ranging from 100 mL to 40 L, these bioreactors offer scalability and efficiency. They feature integrated pH sensors, as well as non-invasive temperature and dissolved oxygen sensors for real-time monitoring and control of critical parameters. Additionally, they eliminate the need for cleaning and sterilization, reducing turnaround time and overall costs.
The sensors transmit data to the Eppendorf DASware® bioprocess control software, which can regulate nutrient feeding, temperature-, DO-, pH-control, gas sparging, and agitation levels to maintain setpoint parameters. This ensures optimal conditions for reproducible growth and increased cultivation efficiency, leading to higher yields.
Furthermore, BioBLU Single-Use Bioreactors can be paired with the DASbox® Mini Bioreactor System for parallel bioprocessing at small-scale, which can streamline process optimization experiments. Researchers at Ncardia®, the Netherlands, utilized this platform to develop a process for scaling up their production of iPSC-derived cardiovascular cells.
Exploring Applications of BioBLU Single-Use Bioreactors
BioBLU Single-Use Bioreactors have demonstrated their applicability for the controlled cultivation of different cell types, including hPSCs, MSCs, and others. Here, we highlight some practical use cases featuring the Eppendorf parallel DASbox Mini Bioreactor System coupled with BioBLU 0.3c Single-Use Bioreactors as a platform to assess and optimize culture conditions to maximize cell growth.
1) Scalable Expansion of hPSCs
The DASbox and BioBLU 0.3c Single-Use Bioreactors platform was used as a platform for optimization of stirring-controlled cell-only aggregate culture of a cord blood-derived hiPSC cell line (hCBiPSC2) for cardiac lineage hPSC differentiation processes. Cells were seeded at 5 x 105 cells /mL (d0) in a stirred suspension fed-batch process and viable cell counts were determined daily. Over a 7-day expansion period, a 4-fold increase in viable cell count was achieved in individual runs over 7 days resulting in up to 2.3 x 106 cells /mL (Figure 2A). The pH and dissolved oxygen (DO) were monitored and maintained at setpoint values throughout the culture period.
Flow cytometry to quantify the expression of pluripotency-associated surface markers TRA 1-60 and SSEA4 at the process endpoint showed most cells retained expression of these markers (84% positivity for TRA 1-60 and 90% for SSEA4) demonstrating that pluripotency was maintained using this cultivation process (Figure 2B).
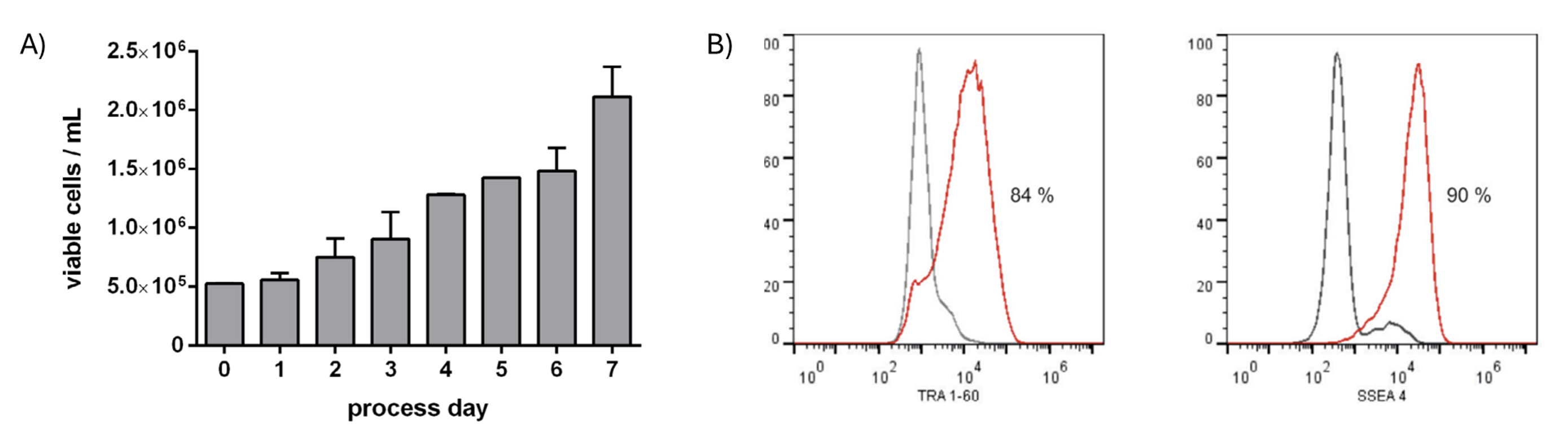
More detailed information is available in this Application Note.
2) Microcarrier Selection for Bone Marrow-derived MSCs Expansion
Two microcarriers, Cytodex type 1 and Cytodex type 3, were assessed as growth surfaces for human bone marrow-derived MSCs (Lonza®, Switzerland).
Cells were culture-expanded in T-175 cell culture flasks for four passages before harvest. MSCs were then combined with either Cytodex type 1 or 3 for parallel experiments using the Eppendorf DASbox Mini Bioreactor System and BioBLU 0.3c Single-Use Bioreactors and evaluated to assess hMSC expansion.
Figure 3A shows the proliferation rate of MSCs was highest using the Cytodex type 1 microcarriers, with a 17.5-fold increase in cell number and a maximum cell density of 1 x 108 cells/bioreactor achieved by day 14, corresponding to 4 x 105 cells/mL. Conversely, on Cytodex type 3 microcarriers, it took 20 days to reach a maximum cell number of 7 x 107 cells/bioreactor, which is an 11.5-fold increase from the initial seeding quantity and corresponds to 2.5 x 105 cells/mL.
Notably, hMSCs expanded on both microcarrier types retained their multipotency and ability to differentiate into osteocytes and chondrocytes (Figure 3B). These findings demonstrate the efficacy of the BioBLU 0.3c Single-Use Bioreactors in supporting hMSC expansion while preserving cellular functionality and differentiation potential.
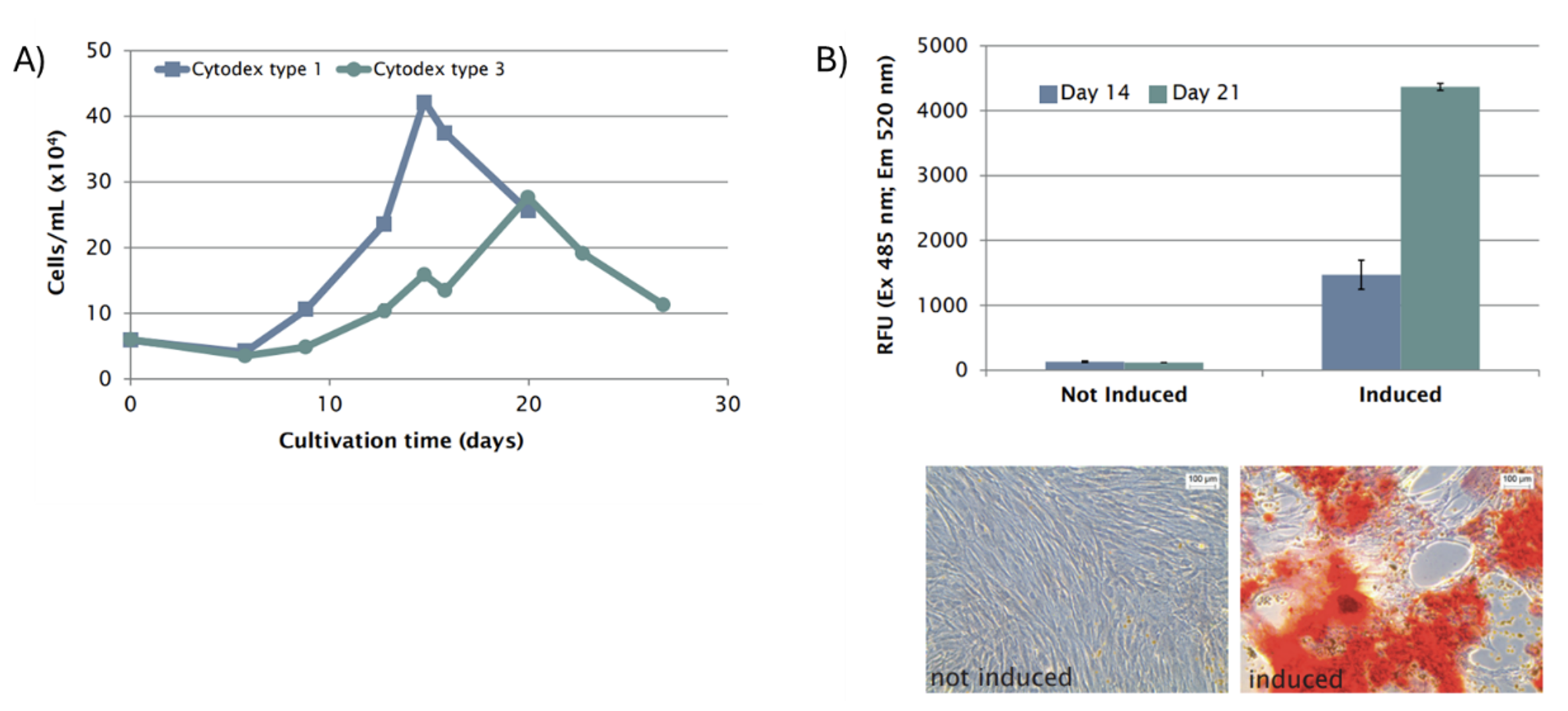
Access to supplemental information about these experiments in this Application Note.
3) Optimizing Long-term Expansion of CD4+ T Lymphocytes
The DASbox Mini Bioreactor System and BioBLU 0.3c Single-Use Bioreactor platform has proven effective in optimizing the long-term expansion of CD4+ T cells, a crucial subset of immune cells vital for adoptive cell therapy (ACT) strategies like CAR-T cell therapy. These primary cells are highly sensitive to their culture environment, which can impact their quality and efficacy in ACT.
The platform was utilized to investigate the impact of oxygen levels on T cell expansion efficiency. Human peripheral blood CD4+ T cells from Lonza were cultured at two oxygen tensions (70% or 20% dissolved oxygen) for 16 days. Regular assessments were conducted for cell proliferation, viability, glucose, and lactate concentrations. Both conditions demonstrated high CD4+ T cell proliferation rates, with a higher fold expansion observed at 20% dissolved oxygen (Figure 4A).
Specifically, an average fold-expansion of 2.35 x 106 and 9.63 x 105 was observed for 20% DO and 70% DO, respectively. Additionally, CD4+ T cell functionality was evaluated by measuring interleukin 4 (IL-4) production. Samples taken at days 10, 17, and 20 were either stimulated with PMA and ionomycin for 24 hours or left untreated before IL-4 levels were determined. Figure 4B illustrates that IL-4 inducibility was maintained in stimulated cells but not in non-stimulated cells.
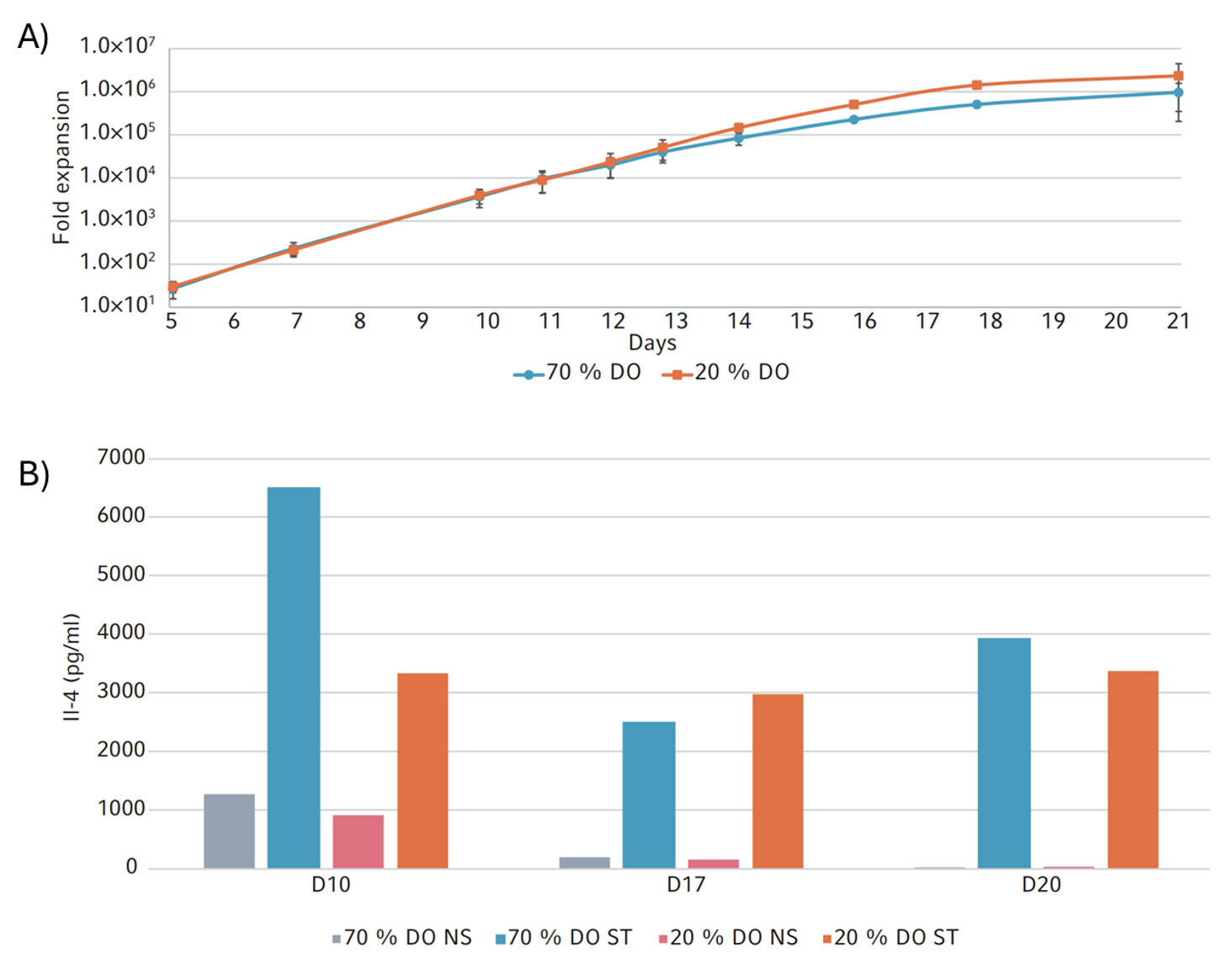
A more detailed summary of these experiments is available in this Application Note.
Partnerships for Success
The Eppendorf Bioprocess Unit, build on decades of experience in upstream bioprocessing, has contributed to the advancement of cell cultivation in stirred-tank bioreactors for many years. The collaboration with our customers is an essential part of the Eppendorf DNA.
One such example is our collaborative work with Dr. Robert Zweigerdt and his team from Hannover Medical School, Germany to increase hiPSC yield grown as cell aggregates in stirred-tank bioreactors for further differentiation into hiPSC-cardiomyocytes. Building on the initial process optimization experiments described in earlier work, our joint efforts led to key innovations, including a specialized 8-blade impeller design tailored for stem cell aggregate cultivation in suspension. Additionally, a retention-filter system was implemented to minimize cell loss during automated perfusion feeding.
These advancements, coupled with the Eppendorf DASbox Bioreactor System and BioBLU Single-Use Bioreactors, yielded remarkable results.
Conclusion
Scalable bioreactor technologies and companion bioprocess control software from Eppendorf can help meet the diverse cell production needs of researchers and overcome manufacturing bottlenecks in the development of advanced therapies. As we have described throughout this article, for commercialization, researchers are evaluating standardization of their cultures and how to scale up efficiently.
The DASbox Mini Bioreactor System and BioBLU Single-Use Bioreactors provide a reliable platform for accelerating cell culture development, process optimization and scale-up for cell types crucial to advanced therapy applications including iPSCs, MSCs, and others. With extensive options for real-time monitoring and control of critical process parameters and scalable bioreactor design, Eppendorf makes it easy to transfer small-scale bioprocess results to larger working volumes. Eppendorf customers worldwide rely on bioprocess solutions and expertise to support their cell and gene therapy research and development.
Learn more about Eppendorf solutions here: www.eppendorf.link/