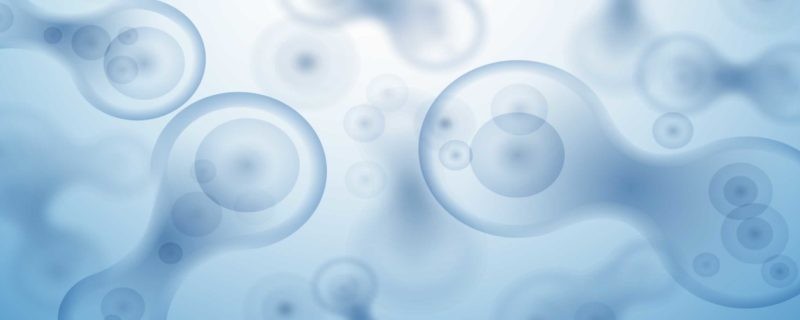
Employing Innovative Platforms for Large Scale Stem Cell Culture
In the first blog in our stem cell series, “New Strategies Key to the Clinical Manufacturing of Stem Cells for Therapeutic Use” three strategies were identified to improve clinical stem cell manufacturing. In part one of the series titled “Optimizing Media for Clinical Manufacturing of Stem Cells for Therapeutic Use,” we examined ways to optimize stem cell culture media for clinical manufacturing including strategies for removing animal-derived ingredients. In Part II of the stem cell series we will look at ways to scale-up manufacturing of stem cells including innovative platforms that enable culture of large numbers of stem cells per batch.
With several new stem cell therapies in development and many showing promising results in clinical studies, it is critical to begin moving stem cell manufacturing from small scale to large scale manufacturing for therapeutic use. Several of these potential therapeutic applications will require billions to trillions of stem cells per lot and manufacturing will need to expand to meet these demands. New technologies have been created to successfully culture large numbers of stem cells in a single batch.
One technology with the potential to meet manufacturing requirements for adult primary cell or induced pluripotent stem cell therapies is the use of “parallel plate” or “multi-plate” bioreactors. These bioreactors incorporate the use of several layers of plates for growing adherent cells. These systems are scalable, allowing the addition or subtraction of layers based on necessary lot size, but ultimately there are limits to the total number of layers that can be run per lot. These bioreactors incorporate varying levels of automation and control over variables including dissolved oxygen, dissolved CO2 and pH and in doing so offer some of the increased scalability of traditional bioreactors while still providing plate based culture. Another advantage is that these systems offer an easier scale up transition for cells used to more traditional lab based plate culture. These systems can be expensive, but offering a smaller footprint and therefore requiring less manufacturing space can offset some of the expense. Other attractive features include a fully closed sterile system and single-use disposable options. Two examples of these systems include ATMI’s Integrity Xpansion and Corning’s Cell Cube.
A fixed bed bioreactor is another possible option for large-scale stem cell culture. In these systems, cells are attached to substrates that are part of the fixed bed. These substrates act similarly to microcarriers, but they do not freely move about, the result is less shear force on cells. Fixed bed substrates can include microfibers made of various substances, hollow fibers, and beads. This type of bioreactor has traditionally been used in monoclonal antibody and recombinant protein production where cells attach to the substrate, then secrete the antibody or protein of interest. In this type of production, product is easily harvested. The primary difficulty with adapting these bioreactors for use in Cell Therapy is that the product are the cells themselves and maintaining good yield during harvest can be a challenge. Recently, there have been adaptations made to these systems to more easily allow the harvest of adherent stem cells. Similar to parallel plate systems, fixed bed bioreactors also provide the ability to control culture conditions including temperature, pH, and dissolved oxygen and they too offer a smaller footprint than stirred-tank bioreactors. One advantage over parallel plate bioreactors is that certain cell types may benefit greatly from having a 3D scaffold. Companies that offer these systems include ATMI’s iCELLis, Beckman Coulter’s Quantum, and New Brunswick’s Fibra-Cel Disk System, and FiberCell System.
Traditional large-scale, stirred tank bioreactors can also be used in stem cell culture by either employing microcarriers or by reprogramming cells for suspension culture. The advantage of stirred tank bioreactors is that they provide a larger vessel for manufacturing and in turn can handle much larger batches at one time. This large-scale production reduces costs by lowering the total number of batches necessary to meet demand.
Microcarriers allow attachment dependent cells to be propagated in bioreactors and have been used successfully in attachment dependent viral cultures for years. With an increase in the use of stirred-tank bioreactors, microcarrier innovations have been necessary to allow adherent cells to be cultured in these conditions. For example, new low-density matrices can be positioned in bioreactors and allow for efficient adherent culture in stirred-tanks. Innovations in microcarriers are now making it possible for adherent cultures to take advantage of the use of bioreactors and optimize their manufacturing. However, there are concerns about using this technology for stem cells, primarily the effect of shear force and agitation on cell health and cell yield. Since the cells themselves are the final product these areas are of particular concern in manufacturing.
Another possibility for the use of large-scale stirred tank bioreactors is to reprogram adherent cells to suspension culture. Researchers have been exploring this new technology. One example is demonstrated through the work of researchers at the University of Calgary, Department of Medicine, who have published two studies on the use of stirred suspension expansion and long-term culture of iPSCs. One study “Derivation of iPSCs in Stirred Suspension Bioreactors” published in Nature Methods described how they were able to reprogram iPSCs derived from fibroblasts to grow in stirred suspension bioreactors instead of adherent culture. The publication makes the point that even at small scale, stirred suspension bioreactors can produce billions of cells in just a few days. Authors believe that “suspension reprogramming could address a major bottleneck in iPSC production and enable efficient reproducible and large scale generation of cells for both research and potentially clinical applications.” Both studies were covered in more detail in a previous blog “Culture and Expansion of Stem Cells in Stirred Suspension Bioreactors Could Provide Key in Large Scale Manufacturing.”
Companies manufacturing stirred tank bioreactors systems include Thermo Fisher Hyclone, GE Wave Biotech, FiberCell, Xcellerex , ZellWerk, ATMI, Refine Technology, AmProtein, Sartorius Stedim, New Brunswick and Biovest. Most commonly stainless steel, stirred-tank bioreactors are used for this application although there has also been an increase in the number of single-use disposable options available.
Single use systems offer another attractive option in the area of stirred tank bioreactors with similar advantages and some additional benefits. They offer similar growth and productivity to stainless steel bioreactors but are more flexible. Single use systems have a faster turnaround time between batches because there is much less cleaning and validation necessary than with stainless steel tanks. Cleaning and validation can be costly and time consuming steps in the manufacturing process and can delay the next manufacturing run.