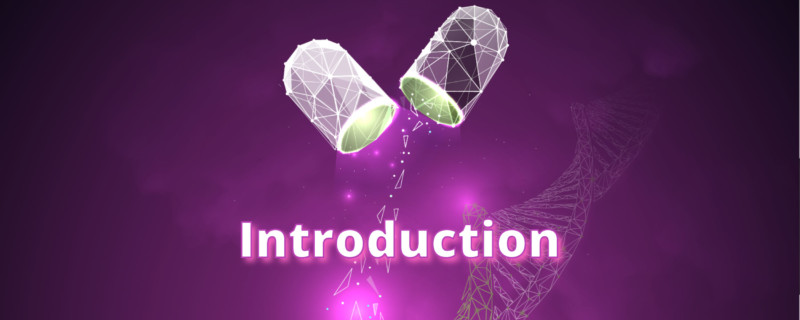
Insights on Successful Gene Therapy Manufacturing and Commercialization
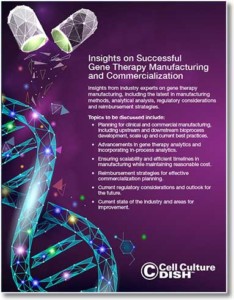
“Insights on Successful Gene Therapy Manufacturing and Commercialization”.
You can download all the articles in the series, by downloading the eBook.
Industry Outlook
The first approved gene therapy study was conducted by the National Institutes of Health (NIH) in 1989 and provided evidence for the first time that human cells could be genetically modified and returned to the patient without harm. Since then, the industry has grown significantly with 22 gene and gene-modified cell therapies approved by regulatory bodies from various countries as of August 2019.1 As of October 2020, six gene and gene-modified cell therapies have been approved for use in the United States, including the latest approval in July 2020 for the gene therapy Tecartus™.
Clinical trials have continued to grow with 352 gene therapy and 452 gene-modified and cell-based immunotherapies in clinical trials globally at the end of last year.2 While the majority of current trials are still in Phase I or Phase II ~91% for gene therapy and ~97% for gene-modified and cell-based immunotherapies, there are nearly 50 trials globally that are in Phase III. This suggests there could very well be dozens of gene therapy product approvals in the coming years. In fact, the FDA expects to see a doubling of new gene therapy applications each year and Scott Gottlieb, the former FDA commissioner, predicted that by 2025, the US would be approving between 10 and 20 gene therapies each year.3
Supporting this clinical and commercial progress has been substantial investment and company growth. Gene and gene-modified cell therapy had its second highest year of investment with $7.6 billion raised in 2019 and there are 496 gene therapy companies working globally.2 However, this success has not been without its challenges. As we cover in this Ebook, gene therapies stand to revolutionize the current healthcare paradigm from patient treatment to drug supply logistics and the best way to execute on the promise of these therapies is still evolving.
How Does Gene Therapy Work?
Gene therapy is the use of a gene-modifying technology to repair, replace or correct damage in the body. For gene therapies to work, genetic material must be introduced into cells to treat disease, which is most effectively achieved using a vector delivery system. Viruses make good vectors for delivering genetic material because they have evolved to do just that—deliver genes by infecting cells. Viral vectors for gene therapy are modified to ensure that they don’t cause infectious disease in the patient. The most commonly used viral vectors for gene therapy include retrovirus, adenovirus, adeno-associated virus (AAV), and lentivirus. While non-viral approaches for delivering gene therapy are being explored, viral vectors are still the most popular approach with two-thirds of the clinical trials to date delivered via viral vector.4
Gene therapies can be delivered by injecting the vector directly into the patient (in vivo) or the vector can be delivered to specific cells harvested from a patient’s blood or tissue collection (ex vivo). The vector is introduced into the patient’s cells using a method called transduction. With ex vivo approaches, the modified cells are subsequently expanded in cell culture before they are injected back into the patient.
Non-viral approaches, offer some advantages including delivery of larger genes, simplified production, and reduced biosafety concerns. However, they have been shown to be less efficient at delivering the genetic material, and in some instances, the therapeutic benefits have been short term. Recent improvements in non-viral methodologies are increasing interest in this approach.
Indications
Perhaps the most talked about gene therapies have been the gene-modified cell therapies for cancer immunotherapy and with good reason. The American Society of Clinical Oncology’s (ASCO) Clinical Cancer Advances 2018 report named adoptive immunotherapy with chimeric antigen receptor T cells (CAR T) as the most important clinical cancer advance of the year. CAR T therapies are both gene therapies and immunotherapies, often called gene-modified cell therapies. In brief, CAR T takes immune cells, called T cells, from a patient then genetically engineers them to express chimeric antigen receptors (CARs) that recognize the patient’s cancer cells. Cells are then infused back to the patient (this process is called adoptive cell transfer, or ACT). These engineered cells circulate in the bloodstream, becoming “living drugs” that target and kill the antigen-expressing cancer cells. With many early successes in clinical trials using CAR T, there is great hope that it can be used to treat a wide variety of blood and solid tumor cancers.
Not surprisingly, cancer is by far the largest category of indications being investigated with 65% of the gene therapy clinical trials in this area. The second most popular category of indications is inherited monogenetic disease with 11.1%, followed by infectious diseases (7%) and cardiovascular diseases (6.9%) rounding out the top four indications.4
Gene Therapy From the Lab to Commercialization
As discussed, gene therapies represent a new paradigm both therapeutically and with respect to how they are managed within the larger scope of healthcare. This has wide ranging impacts on several key areas including: regulatory, manufacturing, patient education, reimbursement, and logistics and distribution. While the process can be modeled on existing biologics, there is a great deal that is different about gene therapies that needs to be addressed.
With clinical success and increased investment from the market, many gene therapy companies are looking toward manufacturing and commercialization of their lead therapies. However, there are still several challenges that must be considered when looking at how these products will be manufactured safely, at appropriate scale, and with reasonable cost. Complicating this task is the fact that there is no “one size fits all” approach as gene therapy products are complex and can be manufactured in a variety of ways, using a variety of vectors and cell lines.
Gene Therapy Manufacturing
Gene therapy manufacturing is a critical part of whether a gene therapy will be successfully commercialized or not. It is important for stakeholders to include process development and manufacturing programs as early as possible in development to keep pace with the rapid movement of gene-based products through the clinical landscape. There is a key balance to be struck between investing too early in manufacturing technology before the product has been fully characterized and running the risk that the manufacturing process doesn’t produce the correct product. On the opposite end, investing too late means attempting to scale up a process that may not meet needs, which could become very expensive and risky. For the purposes of this publication we will focus on viral vector manufacturing.
Manufacturing capacity for the industry is also of great concern, with viral vector-manufacturing capacity estimated to be 1–2 orders of magnitude lower than what is needed to support current and future commercial supply requirements.5 The COVID-19 pandemic has added to strained capacity as some of the COVID-19 vaccine development programs also use viral vectors. Work is being done to reduce the capacity crunch in the short term by adding new manufacturing facilities and additional production capacity. However the most durable capacity increase would come from improving manufacturing practices to increase process productivity. Advancements including engineering cell lines for increased productivity, improving plasmids and constructs and boosting process recovery are all areas currently being explored. For example, current process recovery is quite low, less than 20%. If you increase recovery to 50%, manufacturing requirements can be cut by two and a half.
Gene therapy companies also need to understand what regulatory pathways are available to them based on whether their product fills an unmet need or addresses a serious, life-threatening condition. As such, there are several expedited pathways that may be available for gene therapy developers. If the product is designated under an expedited timeline then this will impact manufacturing timelines and thus needs to be considered during process design, scale-up, qualification, and continuous verification.
Evaluating the Existing Process
Many pre-clinical processes for making viruses are based on academic protocols where scale and quality are not of the highest importance. It is important to determine whether the current process is scalable to clinical and commercial manufacturing and whether quality demands can be met. The Good Manufacturing Practices (GMP) requirements for raw materials, cell substrates and process consumables are much more demanding and the potential long lead times for obtaining those critical items can be challenging. Therefore, evaluating existing processes will help design process development for clinical and commercial manufacturing. It will also help to develop accurate time lines as academic processes that need more changes will take longer to move to a clinical/commercially compatible process.
Scalability
Scale is one of the biggest considerations when designing the manufacturing process. This can be a challenge to achieve, particularly if a product’s approval timeline is accelerated. Determining scale means identifying how much material is needed for clinical trials and ultimately commercial manufacturing. Projected manufacturing scale is calculated by taking the number of patients to be treated per year x dose / desired number of batches per year. So for example, if you plan to treat 200 patients per year at a dose of 1014 viral genomes (vg) per patient, then one needs to understand how much a process and a facility can make in a single batch.
Because product demand and the number of patients treated will usually increase as a product progresses through clinical trial phases and on to commercialization, it is important that the designed process is scalable and that critical quality attributes are well characterized. Therefore, investing in process development and optimization must be appropriately timed, particularly if the product has been granted an accelerated approval process by regulators.
It is also possible that a gene therapy will not need to be scaled-up, for instance in rare monogenic diseases with very low patient populations. In these cases it may be possible to meet all product demands without the need to scale-up. This is why understanding the scale of a disease indication is so critical in manufacturing process design.
In-house or Outsourced Manufacturing
It is estimated that greater than 65% of cell and gene therapy manufacturing is outsourced to contract manufacturers and that gene therapy developers may need to wait up to 18 to 24 months before accessing a Contract Development and Manufacturing Organization’s (CDMO) production capacity.6 This makes it critical for developers to establish a manufacturing plan early in the development timeline by securing either in-house or outsourced manufacturing resources.
The patient population being treated is a key consideration in deciding between in-house manufacturing versus outsourced manufacturing to a CDMO. In conventional medicine, the ratio of the incidence to prevalence of patients for a given disease is expected to be high so that capital expenditures, such as those required to build a manufacturing facility, can be amortized over a number of years. In contrast, for genetic diseases, the ratio of incidence to prevalence population can be quite low. This means that the prevalence population gets treated in the first few years after launch and then a manufacturing facility is only required to treat the incidence population which can be very small for rare and ultra-rare diseases. This means the capital associated with building a manufacturing facility for a single gene therapy has to amortized over just a couple of years after product launch. This can make the business case for building your own facility quite high.
Beyond the expense and dedication of capital to build manufacturing capacity, time and resources required of gene therapy companies and their management must be considered. According to a recent report on the current and forecasted skills demand for the cell and gene therapy industry in the UK, there will be a 112% increase in jobs from 2019 to 2024. There will be a 126% increase in bioprocessing roles including manufacturing, supply chain and logistics, process development and total quality. This increase in the need for skilled employees raises concerns about recruitment or retention required for industry growth. Companies involved in the report also expressed concern that academic courses are not producing industry ready graduates and post graduates.7 To help meet this need, gene therapy training centers have been established. For example, BioCentriq™ is the Cell and Gene Therapy Development and Manufacturing Center and Center of Excellence at New Jersey Innovation Institute (NJII). Biofactory Competence Center (BCC) based in Fribourg, Switzerland is another group that provides practical training on gene therapy manufacturing.
Companies must be able to establish the design and scale requirements for the facility, often with limited certainty as to the scale ultimately required, the potential launch quantities, and market penetration to guide planning. Outsourcing can serve as an effective and economical bridge until greater certainty is gained as to product demand. However, for gene therapy companies who either have difficulty finding a CDMO partner or securing production slots in the timeframe they need, building a small, early phase GMP facility may make sense. There are also unique partnership agreements that can be established in lieu of full outsourcing, for example, a monoplant or condo arrangement.
Ultimately gene therapy companies will have to weigh several factors before deciding whether to outsource manufacturing or manufacture in-house and the appropriate time to implement each option.
Manufacturing Processes
Viral vector systems are by far the most widely used methods to delivery therapeutic gene products because of their infectious nature and ability to introduce specific genes into a cell. In addition to creating a safe and efficacious product, the industry must also look at methods to increase capacity, reduce cost of goods, improve therapeutic efficacy and employ analytics that not only enable process optimization, but also provide information critical to regulatory approval. There is progress on several fronts to improve upon existing methods and some key areas of focus have emerged. These will be covered extensively in the manufacturing articles that follow.
Aseptic Manufacturing Controls
Aseptic processing is stated as “Handling sterile materials in a controlled environment, in which the air supply, facility, materials, equipment and personnel are regulated to control microbial and particulate contamination to acceptable levels.”8 While aseptic controls are commonplace in pharmaceutical manufacturing, aspects of gene and gene modified cell therapies make aseptic processes even more critical.
For viral vector production it is important to understand the level of aseptic control that is built into the process. Aseptic control is greater in processes with closed manufacturing and less in systems with manual, open processes. In addition, it is important to consider whether the vector bulk can be sterilized. For instance, viral clearance is used to some degree in both AAV and Lentivirus processes, which confers a level of viral safety.9 The level to which bulk can be cleared of contaminants and the methods available for that dictate the area with the most risk of contamination.
The level of risk is higher with gene-modified cell therapies because there are typically more open operations in these processes. These cultures often include higher risk, animal derived raw materials as well.8 Furthermore commonly used viral clearance and sterile filtration methods are not possible with these therapies. Thus, a focus on prevention of contamination is the best approach. This means good aseptic techniques, transfer to automated processes and removal of operator handling as much as possible. In addition, sterile filtration of raw materials to remove or inactivate any virus or bacteria prior to use in culture is important.
Regulatory Considerations
Currently there are six approved gene therapies in the US and hundreds of products in the pipeline. The Food and Drug Administration (FDA), has responded to the challenge by hiring more reviewers and rapidly developing the much needed regulatory framework.
However, in 2020 there were regulatory disappointments as well. This included the Food and Drug Administration (FDA) denying approval of BioMarin’s hemophilia A gene therapy. Another setback came when Audentes Therapeutics reported a third death in its halted gene therapy clinical trial for a rare genetic neuromuscular disorder. The three patients who died were all from the high-dose AAV vector cohort. There has been concern around the use of high dose AAV vectors in treatments. Researchers are investigating methods for engineering capsids that would target specific tissues, thus enabling lower dosing requirements (see Upstream Manufacturing article for more details). The takeaway from these setbacks is that there is a need for improvement in purification and analytics and that the FDA will likely be expecting more data as developers and regulators expand their understanding of gene therapy characteristics. A more comprehensive regulatory review will be provided in the regulatory article that follows.
Reimbursement Strategies
Gene therapies introduce new uncertainties for payers. From the payer’s point of view the risk is high for an expensive one-time therapy. This is not surprising if you consider how traditional reimbursement occurs, a payer pays a lower cost for a therapy over a longer period of time. In this model, the payer accrues value on their investment over time. With the gene therapy model, they pay a large cost upfront and the value for this investment isn’t accrued until much later. To complicate matters, if the subscriber leaves a plan the payer has no opportunity to reap the value of their investment.
Since gene therapies are a new type of medicine, there is still uncertainty around performance durability, the hope is that the treatment will be a cure or will last for a very long time, but that has yet to be proven. Smaller plans will have a more difficult time trying to plan for these rare conditions and define how many cases their plan will have to budget for.
The good news is that the vast majority of payers cover and reimburse gene therapies today. They are working with gene therapy developers to create new financing and reimbursement solutions to ensure sustainability for all plan members as payers transition payment models to address the challenges of one-time high cost gene therapy treatments. For specific reimbursement solutions being used by payers today, please see our article on reimbursement.
Supply Chain Security
The traditional biologics supply chain has been developed and refined over many years. It has been vetted to ensure that every aspect of the supply chain has safety measures and redundancies to mitigate any risks. With gene therapies and gene-modified cell therapies in particular, several of these measures are not possible. For instance, gene modified cell therapies are personalized, autologous treatments. This requires not only delivering the therapy to the patient, but also coordination and collection of patient material to the manufacturing location. Furthermore, these activities must be conducted following very tight timelines. For instance, Novartis has stated that their target manufacturing turnaround time from receipt of patient material to return of product is only 22 days. Gilead has stated that the median turnaround time for Yescarta® is 17 days. Unlike traditional biologics, these therapies have no redundancy. They are personalized to the patient; there is no back up and no margin for error.
These therapies are also complex to transport, they require strict temperature controls, product security and must be constantly monitored for compliance. According to a recent article by Cryoport on the cell and gene therapy supply chain, they state that “A shipping system should include the following elements: validation and shock absorption through advanced internal and external packaging that exceeds ISTA standards; state-of-the-art dewar technology and revalidation processes that ensure cryogenic holding times are actively monitored and confirmed for every shipment; active shipment level tracking that is based on redundant GPS, cellular and WiFi networks constantly monitored around the globe; and 24/7/365 support personnel who can intervene in emergencies. No exceptions. These are the standards that must be adopted globally to ensure patient safety and Cell and Gene Therapy (CGT) success.”10 Thus the supply chain logistics must be carefully planned with a great deal of expertise and no room for error.
Provider and Patient Education and Access
It is important that providers and patients have access to the product. This access can be in the form of logistics, for example with cell-based gene therapies, making sure patients can get to a site to collect their cells or tissue and provide infusion of the treatment. It is important that providers understand proper collection and infusion methods. Cost is also a part of access, so securing reimbursement coverage is important. In addition, patients are increasingly becoming advocates of their own treatment and connection with thought leaders and patient advocacy groups can be invaluable in helping to educate the community on the product, as well as working with payers to ensure coverage. Having a commercial group involved early in a company’s evolution can be very beneficial. These professionals can make commercial projections, identify and interact with patient advocacy groups, and establish the framework for a suitable Target Product Profile. Initial and ongoing stakeholder education is a critical part of any commercialization plan.
In Closing
It is clear that gene therapies hold a tremendous amount of promise when it comes to treating disease, but not unlike any emerging therapy there are challenges that are still being overcome. The good news is that recent product approvals and clinical successes have secured investment and resources to improve upon current manufacturing processes and build the supporting infrastructure required to see the industry reach its full potential.
Suppliers have done a good job creating innovative, fit for purpose products to solve the unique challenges that gene therapy products present and there is still more innovation needed. Developers are effectively leveraging lessons learned from monoclonal antibody production to speed the development of new tools to ensure continued success. In looking back, gene therapies have come a long way since that first clinical study in 1989 and looking forward gene therapies have tremendous potential to deliver on the designation – the future of medicine.
Footnotes
-
1. Ma, C., Wang, Z.-L., Xu, T., Z-Y, H. & Wei, Y.-Q. Approved gene therapy drugs worldwide: from 1998 to 2009. Biotechnol Adv 40, 1-13 (2020).
-
2. Alliance for Regenerative Medicine. Advancing gene, cell, & tissue-Based Therapies-ARM annual report & sector year in review: 2019. Accessed Oct. 11, 2020: Retrieved from https://www.alliancerm.org/sector-report/2019-annual-report/
-
3. US FDA (Jan. 15, 2019). Statement from FDA commissioner Scott Gottlieb, M.D. and Peter Marks, M.D., Ph.D., Director of the Center for Biologics Evaluation and Research on new policies to advance development of safe and effective cell and gene therapies Accessed Sept. 9, 2020: Retrieved from https://www.fda.gov/news-events/press-announcements/statement-fda-commissioner-scott-gottlieb-md-and-peter-marks-md-phd-director-center-biologics
-
4. Ginn, S. L., Amaya, A. K., Alexander, I. E., Edelstein, M. & Abedi, M. R. Gene therapy clinical trials worldwide to 2017: An update. J Gene Med 20, 3015 (2018).
-
5. van der Loo, J. C. & Wright, J. F. Progress and challenges in viral vector manufacturing. Human molecular genetics 25, R42-52 (2016).
-
6. Maingi, S. (Mar. 19, 2020). Visualizing the future of contract development and manufacturing for cell and gene therapies. Accessed Sep. 20, 2020: Retrieved from https://www.pharmasalmanac.com/articles/visualizing-the-future-of-contract-development-and-manufacturing-for-cell-and-gene-therapies#:~:text=With%20traditional%20biologics%2C%20approximately%2035,65%25%20or%20more%20is%20outsourced.
-
7. Catapult. UK cell and gene therapy skills demand report 2019. Accessed Oct. 14, 2020: Retrieved from https://ct.catapult.org.uk/sites/default/files/publication/Catapult_01_UK%20Skills%20Demand%20Report%202019_published_v2.pdf
-
8. Parenteral Drug Association (2011). Process simulation for aseptically filled products PDA task force-2011. Accessed Oct. 11, 2020: Retrieved from https://store.pda.org/TableOfContents/TR2211_TOC.pdf
-
9. Barone, P. W. et al. Viral contamination in biologic manufacture and implications for emerging therapies. Nat Biotechnol 38, 563-572 (2020).
-
10. Wilson, P. (Nov. 21, 2019). Avoiding catastrophe: Addressing the supply chain risks in cell and gene therapy development. Accessed Oct. 11, 2020: Retrieved from https://www.cryoport.com/biological-shipping-blog/supply-chain-risks-cell-gene-therapy