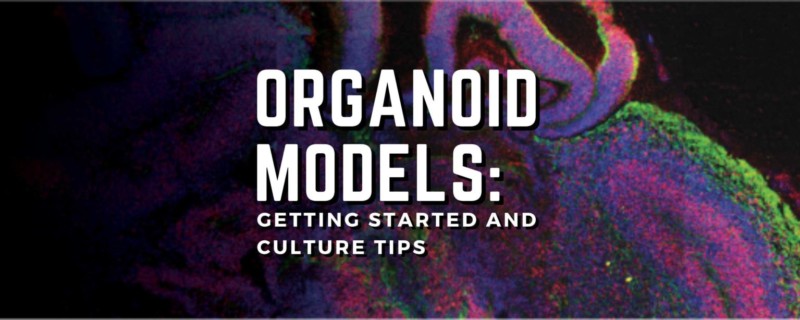
Organoid Models: Getting Started and Culture Tips
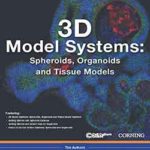
“3D Model Systems: Spheroids, Organoids and Tissue Models”.
You can download all the articles in the series, by downloading the eBook.
An organoid is defined as an in vitro 3D cellular cluster derived from primary tissue (lineage restricted adult stem cells), embryonic stem cells (ESCs), or induced pluripotent stem cells (iPSCs), capable of self-renewal, self-organization, showing similar organ functionality as the tissue of origin. Their similarity in composition and function to or-gans in vivo makes them invaluable 3D models to study organogenesis, disease modeling, and patient-specific therapies. Organoids recapitulate a large number of biological parameters including the spatial organization of heterogeneous tissue-specific cells, cell-cell interactions, cell-matrix interactions, and certain in vivo physiological functions generated by tissue-specific cells within the organoid¹. Organoids bridge a gap between 2D and animal model systems by providing a stable system amenable to extended cultivation and manipulation. As such, researchers have created physiologically relevant organoid models for many organs. That said, working with organoids is not without its challenges. High quality cell culture tools are important to ensuring success when researchers are looking to incorporate organoids into cell culture workflows.
It is well known that cells in living tissues interact with other cells as well as the tissue microenvironment for survival, proliferation, differentiation and migration. The extracellular matrix (ECM) provides biological cues to support cell function as well as physical support for cells to grow and move in 3D. The presence of biological or synthetic scaffolds is important for the formation of organoids as they mimic the ECM for a more “in vivo–like” cell culturing system. Ultimately, the choice of scaffold will depend on the tissue of interest and research application. There are many variables to consider, such as different porosities, permeability, surface chemistries, and mechanical characteristics, which need careful consideration for successful organoid cultures.
Natural Scaffolds
Corning® Matrigel® Matrix
Corning® Matrigel® matrix is an ECM-based natural hydrogel commonly used to form 3D organoids (Figure 1). This reconstituted basement membrane is extracted from Engelbreth-Holm-Swarm (EHS) mouse tumors providing the physical support and chemical cues that resemble the in vivo ECM. Matrigel contains molecules important for cell function, differentiation and attachment (i.e. laminin, collagen IV, heparin sulfate proteoglycan, and nidogen/entactin). Matrigel is often used to generate cancer organoids because the components in the matrix can activate signaling pathways in cancer cells that control angiogenesis, cancer cell motility, and drug sensitivity.
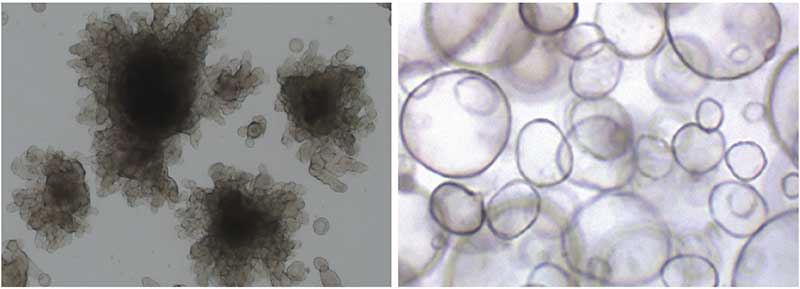
EHS-derived matrices have been extensively published for generating viable and sustainable organoids used in drug discovery and toxicological studies. For a list of recent publications where Matrigel matrix is used for establishment of organoids, please visit www.corning.com/catalog/cls/documents/citations-references/CLS-AN-503.pdf.
An example schematic for cerebral organoids generated from human pluripotent stem cells with Matrigel matrix is shown in Figure 2.
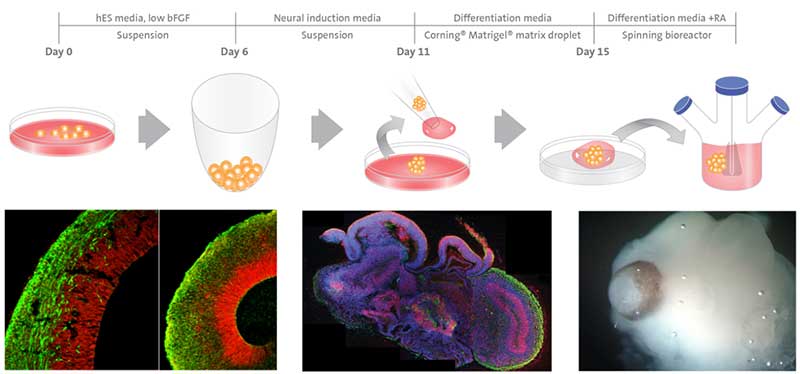
For tips and tricks on using Corning Matrigel matrix, please visit: www.corning.com/worldwide/en/products/life-sciences/resources/webforms/the-ultimate-guide-to-corning-matrigel-matrix.html.
COLLAGEN TYPE I
Corning® Collagen Type I is a natural hydrogel found in stromal compartments in the dermis, tendon, and bone often isolated from bovine skin, rat tail tendon, and human placenta. The type I molecule of collagen is a heterotrimer of 300 nm length and is com-posed of two 1(I) chains and one alpha2(I) chain. It supports in vivo-like 3D growth and differentiation and also interacts with receptors to modulate the expression of genes involved in cell invasion, sensitivity to anti-cancer drugs, cell proliferation, and cell migration. Collagen I has been successfully used to culture intestinal, pancreatic, mammary, brain and salivary organoids.
Sachs et al² found that with collagen I, murine tissue-derived intestinal epithelial organoids aligned and fused to form macroscopic hollow tubes with a single lumen and budding crypt-like domains, more closely recapitulating the native architecture of the small intestine. This is in contrast to traditional Matrigel matrix derived intestinal organoids which form as microscopic single budding cysts. It is postulated that these collagen I-derived tubes may be better for investigating drug effects and development of the intestinal epithelium, where a larger overall size and/or a bigger villus compartment would be advantageous. This also highlights how the choice of scaffold can greatly affect the 3D structure of the resulting organoid.
For answers to frequently asked questions when using Collagen I, please visit: www.corning.com/catalog/cls/documents/faqs/faq_DL_AC_004_Collagen_Products.pdf.
Hydrogels such as collagen I and Matrigel matrix can be used to engineer organoids with precise geometries using novel 3D bioprinting. When mixed with cells, biological inks (bio-inks) can create organoids that reproduce the structure of native tissue by allowing control over the spatial positioning of cells in 3D. Corning Transwell® permeable supports have been successfully used as a bioprinting substrate for a variety of tissue models, including liver and kidney. The microporous surface and the compartmentalized design of the inserts are suitable culture of the bio-printed tissue that can be subse-quently used in drug discovery testing and disease modeling (Figure 3).
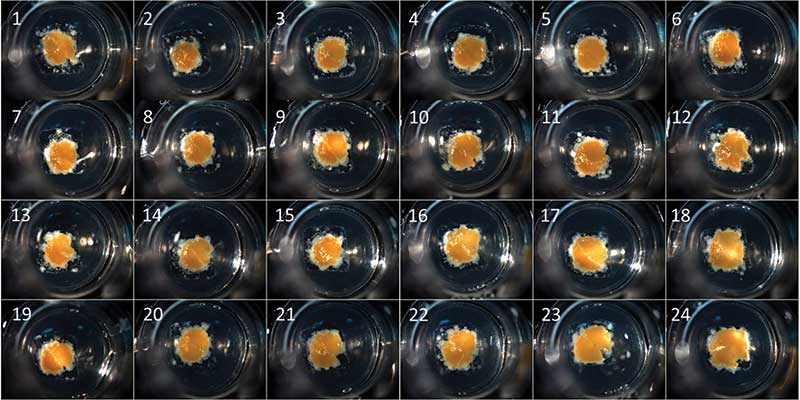
Synthetic Scaffolds
In cases where bioactive compounds, such as growth factors, can potentially interfere with the specific cell behaviors or responses, synthetic hydrogels are an excellent alternative for 3D cell culture applications. Matrigel matrix is animal-derived and generally not well defined with batch-to-batch variability, which can make controlling the ECM environment and downstream applications difficult. To circumvent this, essential components identified in the native ECMs can be incorporated into synthetic polymer scaffolds to produce custom, defined and tunable scaffolds tailored specifically for the organoid system of choice. Synthetic hydrogels are composed of biologically inert, pathogen-free molecules that provide structural support for a variety of cell types. They are simple to process and manufacture making them amenable to large-scale, high-throughput workflows.
CORNING® PURAMATRIX™ PEPTIDE HYDROGEL
PuraMatrix™ is a synthetic peptide mixture capable of self-assembly into a 3D hydrogel with nanometer-scale fibrous structure. When combined with bioactive molecules (i.e. growth factors, ECM components, etc), it creates a defined 3D environment for cells and has been shown to promote differentiation of hepatocytes and neural cells. PuraMatrix™ (RADA-16) also has utility for 3D encapsulation applications, tumor cell migration and invasion, angiogenesis assays and in vivo analyses of tissue regeneration. The transparent nature of this hydrogel makes it easy to visualize samples using standard staining and microscopy techniques. This peptide mixture was found to be superior to collagen I as a scaffold for ovarian cancer cell lines to adhere and migrate in a 3D environment³.
For more information on PuraMatrix™ please visit: www.corning.com/media/worldwide/global/documents/faq_DL_028_Corning_PuraMatrix_Peptide_Hydrogel.pdf.
Organoids representing many different organs allow researchers to better understand developmental processes as well as to establish more accurate disease models. Human diseases that are poorly recapitulated in animal models can be studied with patient-derived organoids. The hope is that organoids could be used as a tissue source for transplantation into patients; however, many obstacles still need to be addressed along the way, such as proper maturation and the lack of vascularization. Stem cell-derived organoids may only recapitulate the first few months of development, but not the stages beyond, therefore, potentially lacking in cell types of interest for re-searchers. In this case, tissue organoids generated from isolated adult stem/progenitor cells or resected fragments of organ tissues (i.e. intestinal crypts, liver, or pancreas ducts) may be more suitable. Currently, the size of the organoids is constrained by the maximum distance of diffusion for nutrients and gas exchange. Larger organoids are desired for certain applications because their increased complexity improves their biological relevance. Several strategies being implemented include co-culturing organoids with endothelial cells to develop vasculature and using microfluidics to generate “organ-on-a-chip” systems (Figure 4) to improve nutrient delivery to the cells allowing larger, more complex organoids to be developed.
While this area of 3D models is relatively new, organoids have emerged as a powerful tool to complement existing animal and cell line models offering unprecedented insight into human development and disease. This opens up innovative approaches to medical research, drug discovery, toxicology testing and for advancing precision and regenerative medicine bearing great promise for the future.
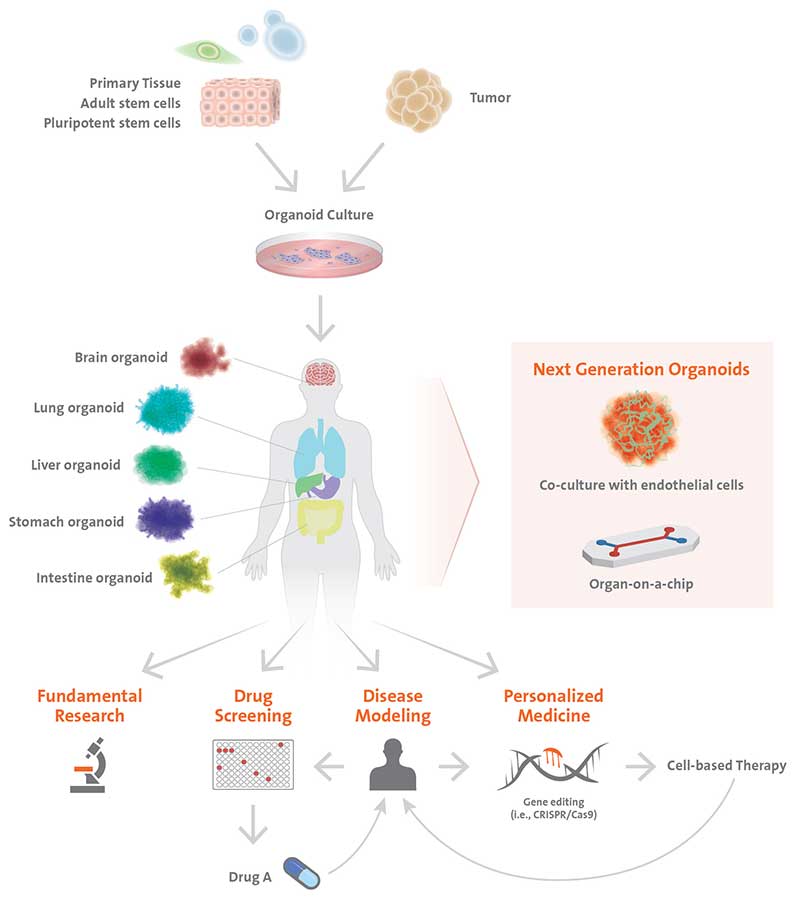
Footnotes
-
1. Yin, X., Mead, B. E., Safaee, H., Langer, R., Karp, J. M., & Levy, O. (2016). Engineering Stem Cell Organoids. Cell stem cell, 18(1), 25–38. doi:10.1016/j.stem.2015.12.005
-
2. Sachs, N., Tsukamoto Y., Kujala P., Peters, P.J., Clevers, H. (2017). Intestinal epithelial organoids fuse to form self-organizing tubes in floating collagen gels. Development, 144(6): 1107–1112. doi: 10.1242/dev.143933
-
3. Yang, Z., & Zhao, X. (2011). A 3D model of ovarian cancer cell lines on peptide nanofiber scaffold to explore the cell-scaffold interaction and chemotherapeutic resistance of anticancer drugs. International journal of nanomedicine, 6, 303–310. doi:10.2147/IJN.S15279