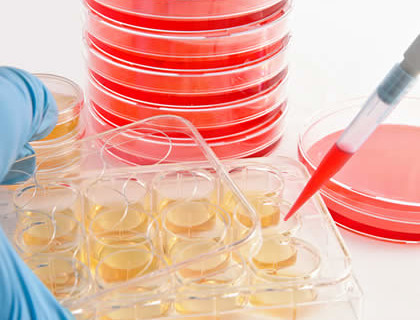
Part II – Key Tools and Technology Hurdles in Advancing Stem-Cell Therapies
This white paper was sponsored by: California Institute for Regenerative Medicine Alliance for Regenerative Medicine – Cell Therapy Catapult and is reprinted in Cell Culture Dish in two parts and with permission.
Authors: Elona Baum, Neil Littman, Morrie Ruffin, Stephen Ward, Kathy Aschheim
Yesterday we posted Part I of this blog covering a Roundtable convened by The California Institute for Regenerative Medicine (CIRM), the Alliance for Regenerative Medicine (ARM), and the Cell Therapy Catapult (Catapult). The Roundtable was convened on June 25, 2013 to define technological challenges and identify potential solutions in three key areas: manufacturing clinical-grade cell products derived from stem cells, characterizing the cell product, and imaging cells after transplantation. This White Paper summarizes the proceedings from the roundtable and highlights key recommendations.
II. Cell-Product Characterization
The ability to characterize diverse properties of a stem cell–based product is essential at all stages of research and commercial development. Cell products are characterized with respect to their identity, purity, viability, and potency; this is critical for establishing lot- to-lot consistency according to CMC requirements. Characterization assays are needed to establish and monitor process components (cell banks, differentiation reagents, scaffolds, and culture systems) and to define process requirements (harvesting and storage, automation, transportation, and expansion), as well as to understand the function of the product in vivo (regeneration, engraftment, integration, potency, mechanism of action, longevity, immunogenicity, and toxicity). A wide array of approaches for designing characterization assays and measuring biomarker expression is already available. These include imaging methods, immuno-assays, biochemical approaches, genomic analysis, deep sequencing, gene-expression profiling, micro-arrays, genetic modification, proteomics, mass spectrometry, flow cytometry, enzyme-activity assays, biosensors, and receptor-binding assays. Given this abundance of technologies, the number of variables and combinations of variables that could be measured in a cell product is essentially unlimited. Determining what to measure requires a thorough understanding of the biology of the cells and of their behavior after transplantation into patients. Because this knowledge is often lacking, the main challenges in product characterization can go well beyond technology to fundamental biological questions about the cells and their clinical effects.
Biological Unknowns
One set of questions relates to cell heterogeneity. Any cell population in clinical development is a mixture of cells that vary in their differentiation stage, lineage identity, and other characteristics.Because the cells are outside of their native environment in conditions that are more or less hostile, they differ from their counterparts in the human body in subtle ways.
- How deeply should heterogeneity be analyzed? For example, with cell therapies based on pluripotent stem cells, it is important to measure both residual undifferentiated cells capable of teratoma formation and contaminating cells capable of forming ectopic tissue. The importance of understanding the potential of cells to produce ectopic tissue was underscored by the clinical hold placed on Geron’s GRNOPC1 phase 1 clinical trial for spinal cord injury, which was triggered by the appearance of cysts in a preclinical study during the enrollment phase of the trial (but prior to patients receiving cells). Ultimately, this issue was addressed by Geron, and the trial was permitted to proceed.
- How does one identify the subpopulation(s) with therapeutic activity? Identifying cell-surface markers of the active subpopulation(s) is a challenge common to many cell therapies.
- What is the optimal stage of cell differentiation to achieve the clinical endpoint?Current protocols for differentiating pluripotent stem cells to specific cell types do not generally produce fully mature cells,and the ability of immature cells to acquire the desired functionality after transplantation must be confirmed. In some cases, cells of a different phenotype to that of somatic cells may be desirable if they have advantageous characteristics, such as increased function or robustness.
A second set of questions concerns interactions between cells and their microenvironment. The niche is an all-important determinant of cell identity and function, and it should be considered not only during cell manufacture but at every subsequent stage in a cell-therapy pipeline. In general, however, the niche is not taken into account in potency assays. Similarly, in the clinic, technologies to evaluate the microenvironment in patients before and after cell transplantation are lacking. In most cell therapies, the vast majority of donor cells die soon after transplantation into humans or animals—clear evidence that the interaction of the cells and the microenvironment is suboptimal.
Additional questions are how to determine a cell product’s mechanism of action and how to bridge the gap between animal models and patients.
For all of these questions, the extent to which they must be addressed in developing potency assays and other characterization assays and biomarkers depends on the particular therapeutic application.As with the manufacturing challenges discussed in Section I, the roundtable participants emphasized that the most effective strategy for thinking through these issues and designing appropriate experiments is to anticipate regulatory requirements and sound development principles and use these as a guide to define a clear pathway to regulatory approval and commercial success as early as possible. The assays used in research might be different from those used in an IND filing, but they should be informed by an understanding of regulators’ expectations. The ultimate goal is assays and biomarkers that serve as reliable surrogates of clinical outcomes.To achieve this goal, it is advisable to first collect as much data as possible and then determine which data are necessary and which are superfluous by searching for correlations with clinical outcomes. The quantities of data being generated by high- throughput technologies are increasing exponentially; storing, exchanging, and mining large data sets represent additional challenges in cell-product characterization.
Summary of Technology Opportunities in Product Characterization
The roundtable participants identified a set of tools and technologies for product characterization that, if developed, would be broadly beneficial to the cell-therapy industry. Table 1 in Section V summarizes these key technology opportunities, which include:
- Sensitive assays to analyze the heterogeneity of cell populations and detect contaminating cells. The problem of contaminating cells is of particular concern for therapies based on pluripotent stem cells as residual pluripotent cells could give rise to teratomas or extraneous tissue. Animal studies suggest that to address the teratoma risk and to provide an appropriate safety margin it may be sufficient to be able to detect ~10,000 residual pluripotent cells. In some cases, single-cell analysis may be necessary.
- Approaches to monitor and manipulate interactions between cells and their niche in vitro and in vivo. Methods for modeling the disease environment in vitro would allow screening for conditions that enhance cell survival after transplantation. Normal, healthy cells may not be the cells that are best able to survive in the disease environment. Possible strategies for manipulating the niche include focused ultrasound, biomaterials or drugs that make the microenvironment more hospitable and/or recruit cells to specific sites. Another useful form of niche analysis and manipulation would be methods for large-scale screening of differentiation protocols. Such approaches would aid the design of better bioreactors.
- Appropriate large-animal models and corresponding same-species cell lines for as many cell types as possible (e.g., embryonic stem cells and induced pluripotent stem cells for large animals). Such models would make it possible to disentangle questions about therapeutic efficacy from problems related to the species difference, such as physiological incompatibilities and immune rejection.
- Companion diagnostics, to stratify patients and determine who is most likely to benefit from a therapy, and prognostic biomarkers, to predict the course of disease and tailor treatment accordingly. Predictors of clinical outcomes should be patient-specific to optimize individual treatment regimens. Companion diagnostics and prognostics have been largely ignored in the cell-therapy industry (in contrast to the rest of the drug industry), with perhaps the exception of the immune Cell Therapy sector.
- Guidance systems to deliver cells in vivo and control homing.
- Sensitive methods for understanding theimmune response to allogeneic cells and effective strategies to induce tolerance.
- More nuanced ways to monitor clinical efficacy and outcomes. Conventional measures may not capture quality-of-life improvements.
- Suitable methods for detecting chromosomal mutations at higher resolution than by karyotyping (copy-number-variation analysis, deep sequencing, etc.) and for assessing acceptable levels of mutation based on functional consequences.
- High-throughput electrophysiology assays.
- Computational tools for data analysis,representation, and management. It may be possible to adapt tools that already exist in other industries, such as biomedical engineering and the financialindustry. As an example, Spotfire, used in the financial industry, has been widely adopted by pharmaceutical companies as an analytics software.
III. Imaging
Given the current technological challenges of imaging transplanted cells in patients, many of the roundtable participants questioned whether it is worthwhile to attempt to incorporate in vivo imaging into clinical protocols. The main drawback of current technologies is low sensitivity and spatial resolution: depending on how well cells are labeled, 600–5,000 cells/voxel for MRI with iron oxide labeling; 20,000–50,000 cells per voxel for MRI with fluorine labeling;and 100,000–1,000,000 cells per voxel for SPECT and PET. There are detection limits and limits in image resolution that cannot be exceeded because of physical constraints such as permissible acquisition times(the time that patients can spend in a scanner), permissible doses of contrast agents, and contrast-to-noise levels (i.e.,the signal intensity of the image). These limitations will vary with the technique used to acquire images, with the magnetic field strength for MRI (i.e., 1.5 vs 3 vs 7 Tesla scans), and with the location in the body being scanned. Longer acquisition scans in higher magnetic-field-strength systems increase the sensitivity to fewer numbers of labeled cells in tissues. A central issue is one of contrast-to- noise; the resolution may not be as important if there is a large amount of signal from the labeled cells.
Another consideration is that the transplant site in the body may not be amenable to certain imaging techniques. For example, in a glioma clinical trial testing neural stem cells labeled with the super paramagnetic iron oxide contrast agent ferumoxytol (Feraheme), of 50 million transplanted cells, only about 1,000 were detected by MRI, because (iron-containing) blood near the tumor confounded the interpretation, making it difficult to distinguish the Feraheme-labeled cells in the area of bleeding around the tumor.
Despite these challenges in in vivo imaging, the roundtable participants stressed that the ability to image cell grafts non-invasively at high-resolution for months or years after transplantation would offer many potential benefits. Understanding the cells’ survival, biodistribution, pharmacokinetics, and pharmacodynamics could help bridge the gap between patient outcomes and treatments.This information would be invaluable in efforts to discover predictors (both imaging-based and non-imaging-based) of clinical outcomes. For example, in Parkinson’s patients, donor dopaminergic neurons have been quantitatively imaged by [18F]fluorodopa PET for many years after transplantation, providing crucial information to correlate with efficacy.
Imaging data would also be useful in optimizing treatment protocols for individual patients (e.g., by determining whether the patient needs repeat dosing). Technical problems during cell delivery could be distinguished from poor cell engraftment. Data on cell biodistribution would aid safety monitoring, such as ensuring that donor cells administered intravenously are not accumulating at ectopic sites such as the lung. In addition to imaging the transplanted cells themselves, it would be desirable to monitor the graft site to assess the patient’s immune response or the status of the tissue after the graft has disappeared. Finally, imaging of transplanted cells and their niche could be combined with existing imaging approaches capable of monitoring organ function and other clinical outcomes.
Will it be necessary to track cell products in vivo and, if so, at what resolution? There is no generic answer to these questions,and it is best to evaluate them for specific therapies from the perspectives of commercialization costs, regulatory approval, and clinical practice. Even if in vivo imaging was possible, the prospect of following thousands of patients for many years would likely be very expensive. In the context of clinical practice, the need for imaging will depend on the therapeutic indication, the cell type, and the window of efficacy. For example, imaging would be more useful for cells that take longer to show efficacy or that persist long-term in vivo (months to years) and less useful for cells that have short-term effects.
Summary of Technology Opportunities in Imaging
The roundtable participants identified a set of tools and technologies for imaging that, if developed, would be broadly beneficial to the cell-therapy industry.Table 1 in Section V summarizes these key technology opportunities, which include:
- Improved PET reporter-gene methods. This is a generic method for monitoring several properties of transplanted cells (biodistribution, differentiation, proliferation) and allows long-term monitoring, but it is less sensitive than traditional PET with contrast agents. In addition, random genomic integration of reporter genes raises safety concerns, although these may be addressable by footprint-free methods for site-specific integration (zinc- finger nucleases, phiC31 integrase, etc.).
- New MRI, PET, and SPECT contrast agents to complement the few that been approved (e.g., (111)In-oxine, technetium-99m).
- Label-free imaging approaches based on cell type–specific markers. HLA markers may be useful for distinguishing donor and recipient cells.
- Cell-surface markers of specific tumor types for anti-cancer cell therapies.
- Multimodality, multitracer imaging methods.
- For therapies based on pluripotent stem cells, imaging methods to screen for teratoma formation.
- Imaging methods to support potency assays.
- Live imaging of single cells to screen differentiation protocols.
- Imaging approaches for companion diagnostics and prognostics.
- Methods for monitoring cell migration in vivo.
IV. Public and Private Initiatives
The US cell-therapy industry is working to address some of the technology challenges discussed in this White Paper. The International Society for Cellular Therapy(1) (ISCT) has provided recommendations on the potency assay.(2) ARM and ISCT are developing four white papers covering cell content/viability; cell identity and purity; immune modulation; and angiogenesis. Furthermore, ARM is engaged in discussions with the White House Office of Science and Technology Policy on a ‘Grand Challenge’ for regenerative medicine that will address a number of the manufacturing issues outlined here. Many of these issues will also be discussed in an FDA roundtable on cell-therapy product development and standards to be held on October 7, 2013. Other important initiatives include the work of Production Assistance for Cellular Therapies, funded by the National Heart, Lung, and Blood Institute,to promote translational research and manufacturing best practices in cell therapies for heart, lung, and blood disease. To address the problem of ‘big data,’ the National Institutes of Health recently announced a $96 million program over four years to support six to eight centers of excellence as part of its ‘Big Data to Knowledge’ initiative, which will fund research, training, and tools needed to exploit large biomedical data sets.
Various programs underway in the UK might provide models for the US stem-cell community. Advanced Therapy Medicinal Products Manufacturing Community(3) is a grassroots effort to publicize best practices for cell-therapy manufacture and to share non- commercial information (such as researchers’ experiences and an ‘Angie’s list’ of reagents). The British Medical Council funded five laboratories for cGMP derivation of hESC lines, and the Medical Research Council has recently established four Regenerative Medicine Platform hubs to perform cohesive early translational work, including manufacturing and characterization. The Healthtech and Medicines Knowledge Transfer Network(4) organizes pre-competitive workshops and committees targeting issues such as the supply chain, storage, and shipping of cell products. The UK BioIndustry Association(5) supports a Quality by Design tool kit for small- and medium-sized enterprises through its Manufacturing Advisory Committee website. Catapult aims to bridge the translational gap by providing technical, clinical, business, and regulatory expertise to stem-cell researchers in order to‘de-risk’ early-stage assets and develop them to a point at which they become attractive to outside investors.
Some of the roundtable participants noted that an important way to build on these efforts would be for the cell-therapy industry to reach out to organizations and scientists in other industries and fields (e.g., The International Conference on Harmonization of Technical Requirements for Registration of Pharmaceuticals for Human Use)(6). Truly disruptive technologies may emerge through collaborations with scientists in areas such as mathematics and systems biology (to develop predictive models of clinical outcomes), engineering and materials science (to optimize cell manufacture), electrical engineering (to create synergies between Cell Therapy and microelectronics), and computational biology (to master data analysis and management).
V. Conclusion
The goal of this roundtable was to discuss key technology hurdles that are slowing the commercialization of stem cell–based therapies and to propose potential solutions (summarized in Table 1). The participants agreed that a publicly accessible Tool Box providing validated, customizable solutions relevant to a large proportion of commercialization efforts would catalyze rapid progress across the cell-therapy industry. In highlighting areas of common interest, the Tool Box would also promote constructive
interactions between industry and academic researchers. Building such a Tool Box cannot be accomplished without substantial financial investment, and support by all stakeholders is urgently needed. Potential routes to developing tools for the Tool Box include industry involvement through pre-competitive consortia, initiatives by organizations such as ARM and Catapult, participation by funding agencies, vendor-supported certification programs, and competitions. In particular, funders such as CIRM might consider how to facilitate the establishment of pre-competitive collaborations among companies to identify and develop technologies of mutual interest.
Summary of Technology Opportunities in Product Manufacturing | |
---|---|
1 | Methods for expanding pluripotent and differentiated cells to large numbers |
2 | Methods for making the growth microenvironment more hospitable to cells |
3 | Methods for enclosed volume reduction without centrifugation |
4 | Methods for improving yield during cell isolation |
5 | Small molecules to replace growth factors and cytokines |
6 | Synthetic matrices to replace biological ones |
7 | Methods to provide cells in final formulation media |
8 | Other strategies to better control the purity and identity of the cell product |
Summary of Technology Opportunities in Product Characterization | |
---|---|
1 | Sensitive assays to analyze cell heterogeneity and detect contaminating cells |
2 | Approaches to monitor and manipulate interactions between cells and their microenvironment |
3 | Large-animal models and corresponding same-species cell lines |
4 | Companion diagnostics and prognostic biomarkers |
5 | Guidance systems to deliver cells in vivo and control homing |
6 | Sensitive methods for understanding the immune response to allogeneic cells and tolerance induction strategies |
7 | Methods to monitor clinical efficacy and outcomes |
8 | Methods to detect chromosomal mutations at high resolution |
9 | High-throughput electrophysiology assays. |
10 | Computational tools for data analysis, representation, and management |
Summary of Technology Opportunities in Imaging | |
---|---|
1 | Improved PET reporter-gene methods |
2 | New MRI, PET, and SPECT contrast agents |
3 | Label-free imaging approaches |
4 | Cell-surface markers of specific tumor types |
5 | Multimodality, multitracer imaging methods |
6 | Methods to screen for teratoma formation |
7 | Imaging methods for potency assays |
8 | Live imaging of single cells to screen differentiation protocols |
9 | Imaging approaches for companion diagnostics and prognostics |
10 | Methods for monitoring cell migration using reporter-gene imaging strategies |
Footnotes
-
1. celltherapysociety.org
-
2. Bravery et al., Potency assay development for cellular therapy products: an ISCT review of the requirements and experiences in the industry, Cytotherapy 15, 9–19(2013).
-
3. atmpmanufacture.org
-
4. connect.innovateuk.org/web/healthktn
-
5. blog.bioindustry.org
-
6. ich.org