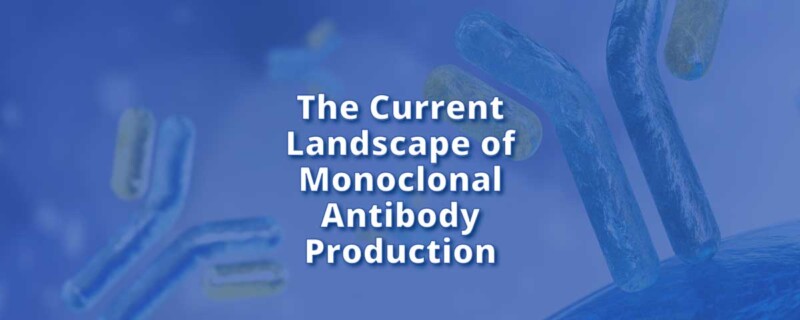
The Current Landscape of Monoclonal Antibody Production
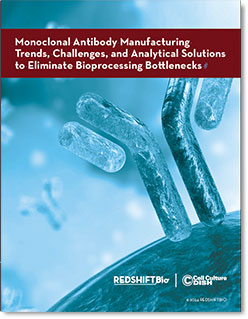
This article was originally published in the eBook
Monoclonal Antibody Manufacturing Trends, Challenges, and Analytical Solutions to Eliminate Bioprocessing Bottlenecks.You can download all the articles in the series, by downloading the eBook.
Bioprocessing technologies to produce therapeutic monoclonal antibodies (mAbs) have evolved tremendously since the first licensed mAb product was introduced to the market in 1986. Early mAb production processes were relatively inefficient and generated titers well below 1 g/L1.
Over the past three decades, the field has evolved significantly, leading to steady improvements in biomanufacturing efficiency and productivity. Along the way, we have seen improved cell lines, optimized culture media and feeds, intensified processing, and modernized purification platforms have collectively increased mAb titers into the gram-per-liter (g/L) range and beyond2,3.
As the importance and demand for mAbs continues to grow, innovations and new technologies in this space will be needed to keep pace. Key drivers such as speed to-market, productivity, and cost-efficiency continue to push the boundaries of current mAb production processes. In this article, we will discuss the current landscape of bioprocess technologies and explore industry trends and advancements that have contributed to enhanced efficiency, cost-effectiveness, and accelerated production timelines.
Data-Driven Decision Making
In this era of data-driven decision-making, advanced analytics, particularly through Process Analytical Technology (PAT) as part of Quality by Design (QbD) methodologies, play a crucial role in guiding manufacturing decisions from cell line development to downstream purification. A typical mAb has 15–20 quality attributes that affect its efficacy and stability, including potency, aggregate levels, variant profile, and glycosylation4. Monitoring these attributes involves a suite of analytical tools; however, many of them originated in the research laboratory and are not well-suited for the bioprocessing environment.
The need for rapid and precise measurements, particularly during upstream processing, has given rise to innovative PAT tools that leverage established technologies like high-performance liquid chromatography (HPLC) and mass spectrometry (MS) that are designed specifically for the bioprocessing space4. Perhaps the most notable shift in mAb manufacturing has been the increased speed of analysis—time-to-data has improved dramatically.
One noteworthy addition to the toolbox is the RedShiftBio HaLCon Protein Analyzer, offering rapid, at-line antibody titer measurements for process monitoring and control that extend across the entire bioprocessing workflow. These next-generation analytical tools can help support PAT initiatives by allowing for real-time adjustments to process variability that help maintain a consistent Critical Quality Attribute (CQA) profile in the final product. Access to rapid and continuous data on critical parameters during various stages of bioprocessing contributes to a more streamlined and data-driven approach to process optimization.
Current State of Upstream Bioprocessing
A variety of mammalian expression systems are utilized for biologics production, but of these, Chinese hamster ovary (CHO) cells dominate the market, producing 70% of biologics and nearly all mAbs5. CHO cells are favored for their ease of genetic manipulation, resistance to human viral infection, ability to perform complex human-like post-translational modification, suitability for large-scale industrial culturing, and history of regulatory approval.
Cell Line Development
Developing stable CHO cell lines for efficient therapeutic protein production involves transfecting host cells with a gene for protein expression, amplifying and selecting clones based on stability and productivity, and finally, choosing the most productive clone—a process that is time-consuming and iterative. Advances in genetic engineering and cell line development methods have enabled more targeted approaches to improve cell productivity.
Since the 2010’’’s, the emergence of omics tools and rational design have contributed to the deeper understanding of cellular characteristics and identity profiles that optimize production5,6. Once omics analysis identifies targets that influence clone productivity, these characteristics can be engineered into cell lines to create stable, high-performance clones. With the development of CRISPR/Cas9 technology, it is possible to precisely engineer host cell lines that are stable and can produce proteins with the desired quality and productivity.
Another avenue for further development is the potential use of alternative expression systems such as bacteria, insect, yeast, and plant cells that can produce antibodies with even higher productivity while preserving glycosylation patterns that are compatible with the human immune system3. These developments have the potential to take the field beyond the current state-of-the-art production in CHO cell culture, yet more work is required to fully evaluate these systems for large-scale biopharmaceutical production processes.
Cell Culture Optimization
To address safety concerns related to prion and viral contamination, serum-free, protein-free, and chemically defined media formulations have replaced serum-containing media in mammalian cell biomanufacturing. Media development, in conjunction with cell line optimization, is a key factor in improving productivity and growth behavior of cells. Media components can have dramatic effects, both positive and negative, on productivity, growth and protein quality. Design of Experiments (DoE) and high-throughput methods are typically employed to assess component concentrations and interactions to identify media components that drive both productivity and desired quality profiles for each monoclonal antibody product7. The availability of scaled-down bioreactor systems coupled with at-line and operator-independent analytics platforms like the HaLCon has facilitated efficient testing of various media formulations, enabling more rapid identification of optimal conditions for cell growth and antibody production.
Spent media analysis is a common practice for optimizing formulations and developing feeding strategies in fed-batch systems. Dynamic Flux Balance Analysis (DFBA) is a new approach, which looks at the relationship between media supplementation, feeding strategies, increased product yield, extended growth phase, and higher cell density7.
Bioreactor Design
Another trend in bioproduction has been the implementation of single-use bioreactor systems, offering benefits like lower capital investment, reduced cost of production, enhanced flexibility, and improved end-product quantity and quality. Improved bioreactor technologies, featuring improved oxygen transfer, mixing, and nutrient distribution, create controlled and efficient environments for cell growth and high-yield antibody production8.
The biopharmaceutical industry employs various cultivation modes such as batch, fed-batch, and perfusion for biologics manufacturing. In perfusion culture, cells are continuously supplied with fresh culture media while spent media and byproducts are removed from the bioreactor. This process allows cells to remain in the bioreactor for an extended period, in contrast to traditional batch or fed-batch cultures where feed media changes are intermittent. Advantages of perfusion include greater consistency in cell viability, higher cell densities, and volumetric productivity. The integration of real-time or in-line monitoring techniques for critical parameters like cell density, viability, and antibody titer, alongside data analytics and process control strategies, provides ongoing insights into the culture’s health, and enable bioprocess engineers to make real-time decisions to optimize the timing for cell harvest to maximize product yield9.
Downstream Processing Bottlenecks
Upstream process intensification advancements have resulted in higher cell densities and antibody concentrations of 10 g/L and higher. This efficiency has introduced new challenges and bottlenecks in downstream processing due to increased product and contaminant concentrations. Downstream antibody processing is typically a platform approach involving Protein A affinity chromatography, followed by additional chromatography steps for polishing and viral clearance, and final formulation and filling. While many of the workhorse Protein A resins used for capture have dynamic binding capacities (DBC) in the 30-45 g/L range, industry interest has grown in alternative chromatographic methods having higher capacity, throughput, and reduced cost10.
Alternatives to Protein A
The growing diversity of antibody-based therapies has led to the emergence of new affinity resins designed to bind to non-Fc-containing fragments or resins with better dynamic binding capacity, milder elution conditions, and resistance to NaOH for clean-in-place operations11. Bioengineers are also exploring various alternative purification technologies, such as Protein G and Protein L chromatography, in addition to non-affinity methods like cation exchange, hydrophobic interaction, mixed-mode chromatography, as well as precipitation and crystallization12. Despite ongoing advancements, Protein A resins are expected to remain the gold standard in monoclonal antibody purification for the foreseeable future. However, the increasing diversification of antibody products and growing cost pressures may introduce new dynamics that warrant a change from the status quo.
Continuous Manufacturing
Continuous biomanufacturing is gaining attention for its potential to improve efficiency, reduce costs, and streamline the production of antibodies. It requires end-to-end integration of all the upstream and downstream unit operations, which occur continuously rather than in distinct batches. This approach offers potential advantages such as increased productivity, reduced footprint, cost-savings, and flexibility in responding to market demands. The benefits of continuous processing have been shown for numerous industries including the small molecule manufacturing
sector7,13.
Continuous operation will amplify the demand for convenient and reliable instrumentation for inline monitoring, and emerging PAT tools can play a catalytic role in accelerating the transition from batch to continuous mAb manufacturing by enabling real-time monitoring and control throughout the entire process13,14. Automation and digital strategies could be solutions to overcome the challenges and enable the continuous manufacturing concept, reduce process variability, and lower production costs8. Fully integrated end-to-end continuous processes in this space are not yet a reality in commercial manufacturing although research and development of such systems is ongoing and has demonstrated feasibility7.
Digital Approaches to Process Development
Advancements in PAT marked by the introduction of new technologies along with the development of comprehensive data libraries, deep learning algorithms, and artificial intelligence (AI) are paving the way for the integration of digital approaches into bioprocessing8. For example, in-silico process modeling provides opportunities to accelerate cell line development allowing for rapid optimization of attributes from potency and selectivity to manufacturability6. Digital twins are another approach that holds promise for reducing costs, accelerating speed-to-market, and enhancing post-approval optimization. A digital twin is a dynamic, real-time digital replica of the physical production process. It incorporates PAT and other monitoring tools to pinpoint process bottlenecks, define crucial engineering goals, and identify operational strategies that enhance the reliability and productivity of their physical counterparts7.
Into The Future
The current landscape of antibody production continues to evolve alongside industry growth, with progress seen in upstream processes such as cell line development and media optimization, as well as innovative downstream purification strategies and the integration of cutting-edge analytical tools like RedShiftBio’s HaLCon Protein Analyzer. These innovations enhance productivity, reduce costs, and help accelerate speed-to-market of the next generation of antibody therapeutics. With their targeted specificity and therapeutic potential, mAbs are offering new therapeutic solutions across various medical conditions from cancer treatment to infectious diseases to autoimmune disorders, making them an important part of the future of healthcare. Continued improvements, like the ones we have discussed here, to the current performance of antibody production processes will be critical to increase patient accessibility, affordability, and sustainability.
This panel discussion was originally published in the eBook
You can download all the articles in the series, by downloading the eBook.
Footnotes
-
1. Kelley B. Industrialization of mAb production technology: the bioprocessing industry at a crossroads. MAbs. 2009;1(5):443-452. doi:10.4161/mabs.1.5.9448
-
2. Stanton D. The Future of Monoclonal Antibody Manufacturing: Incremental Improvement or Industrial Revolution? BioProcess International Book Series. 2018. http://www.bioprocessintl.com/wp-content/uploads/2018/05/16-5-eBook-MAbs.pdf Accessed November 9, 2023.
-
3. Shukla AA, Wolfe LS, Mostafa SS, Norman C. Evolving trends in mAb production processes. Bioeng Transl Med. 2017;2(1):58-69. Published 2017 Apr 3. doi:10.1002/btm2.10061
-
4. Gyorgypal A. Emerging Technological Landscapes in Biomanufacturing and Monoclonal Antibody Screening. Technology Networks. Published: June 6, 2022. Accessed November 9, 2023. https://www.technologynetworks.com/biopharma/articles/emerging-technological-landscapes-in-biomanfacturing-and-monoclonal-antibody-screening-361921
-
5. Savelieff M. Advances in Cell Line Development To Enhance Biotherapeutic Production. Technology Networks. Published: December 14, 2022. Accessed November 9 ,2023. https://www.technologynetworks.com/biopharma/articles/advances-in-cell-line-development-to-enhance-biotherapeutic-production-368347
-
6. Tihanyi B, Nyitray L. Recent advances in CHO cell line development for recombinant protein production. Drug Discov Today Technol. 2020;38:25-34. doi:10.1016/j.ddtec.2021.02.003
-
7. McDonnell S, Floyd Principe R, Soares Zamprognio M, Whelan J. Challenges and Emerging Technologies in Biomanufacturing of Monoclonal Antibodies (mAbs). In: Villarreal-Gómez LJ, ed. Biotechnology - Biosensors, Biomaterials and Tissue Engineering Annual Volume 2023. IntechOpen, 2023. https://www.intechopen.com/chapters/84864 Accessed November 9, 2023.
-
8. Mirasol, F. A Look into Biologic Scale-Up Strategies. BioPharm International 2023; 36 (8), 15–18.
-
9. Valerie I. Collins, Marisa Pisani, David J. Sloan, and Richard Huang. HaLCon, a Fit for Purpose, Platform Method for Rapid Antibody Concentration Measurements from Cell-Free Culture Media. Poser presented at Bioprocessing Summit; August 14, 2023; Boston MA. https://www.redshiftbio.com/resources/halcon-a-fit-for-purpose-platform-method-for-rapid-antibody-concentration Accessed November 9, 2023.
-
10. Jackewitz A. Protein A Alternatives: Mixed Mode Resins For High Throughput mAb Feedstocks. TOSOH Bioscience LLC. https://www.bioprocessonline.com/doc/protein-a-alternatives-mixed-mode-resins-for-feedstocks-0001 Accessed November 9, 2023.
-
11. DePalma A. Alternatives to Protein A Capture Chromatography. BioCompare. Published July 21, 2022. Accessed November 9, 2023. https://www.biocompare.com/Editorial-Articles/588079-Alternatives-to-Protein-A-Capture-Chromatography/
-
12. Gronemeyer P, Ditz R, Strube J. Trends in Upstream and Downstream Process Development for Antibody Manufacturing. Bioengineering (Basel). 2014;1(4):188-212. Published 2014 Oct 1. doi:10.3390/bioengineering1040188
-
13. Maruthamuthu MK, Rudge SR, Ardekani AM, Ladisch MR, Verma MS. Process Analytical Technologies and Data Analytics for the Manufacture of Monoclonal Antibodies. Trends Biotechnol. 2020;38(10):1169-1186. doi:10.1016/j.tibtech.2020.07.004
-
14. Khanal O, Lenhoff AM. Developments and opportunities in continuous biopharmaceutical manufacturing. MAbs. 2021;13(1):1903664. doi:10.1080/19420862.2021.1903664