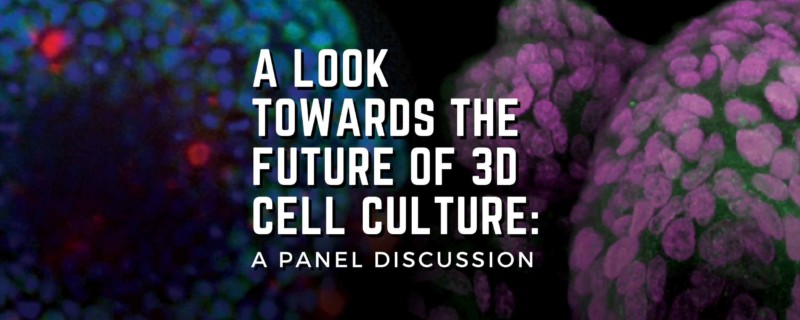
A Look Towards the Future of 3D Cell Culture – A panel discussion
Podcast: Download (Duration: 20:12 — 27.8MB)
Subscribe Here: Apple Podcasts | Spotify | RSS | More
Subscribe to the Cell Culture Dish Podcast on: iTunes | Google Play
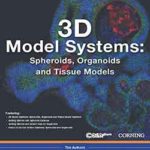
“3D Model Systems: Spheroids, Organoids and Tissue Models”.
You can download all the articles in the series, by downloading the eBook.
Introduction and Overview
Debbie King
Researchers have used 2D cell culture since the early 1900s, but we know that growing cells on planar surfaces have some drawbacks. Cells grown in
vitro in 2D space don’t behave like cells found in vivo. They lack critical cell-cell and cell-matrix interactions that drive their form, function and response to external stimuli. This limits their prognostic capabilities. More recently, 3D cell culture techniques have become popular because the cell morphology, interactions and tissue-specific architecture more closely resembles that of in vivo tissues. Spheroids, organoids and more complex 3D tissue systems, such as ‘organ-on-a-chip’ are examples of 3D cultures used by researchers to model native tissues.
Spheroids are simple, widely used 3D models that form based on the tendency of adherent cells to aggregate and can be generated from many different cell types. The multicellular tumor spheroid model is widely used in cancer research.
Organoids are more complex 3D aggregates, more like miniaturized and simplified versions of an organ. They can be tissue or stem cell-derived with the ability to self-organize spatially and demonstrate organ-specific functionality.
More complex yet, are technologies like organ-on-a-chip, which is a multi-channel 3D microfluidic cell culture system that mimics whole organ function with artificial vasculature. Cells are cultured in continuously perfused micrometer-sized chambers that recreate physiologically relevant levels of fluidic sheer force to allow for gas, nutrient and waste transport to the cell just as is observed in vivo vascularized tissues.
How are spheroids impacting cancer research and what do you see as future applications for the technology?
Audrey Bergeron
Spheroids can be an improved model for cancer in the lab compared to standard 2D cell culture. When cancer cells are cultured as spheroids, they are able to maintain the shape, polarity, genotype, and heterogeneity observed in vivo (1). This allows researchers to create models that are much more reflective of what’s going on in the body. For a simple example, if you think about drug penetration into a 2D monolayer of cells it’s completely different from drug penetration into a solid tumor. In a 2D monolayer each cell is exposed to the same concentration of drug whereas in a spheroid, like a solid tumor, there are gradients of drug exposure.
More and more we’re seeing researchers move away from cancer cell lines and move more toward specialized cancer models such as patient derived models. The hope here is to find the appropriate therapies for each individual patient.
(1) Antoni, D., Burckel, H., Josset, E., & Noel, G. (2015). Three-dimensional cell culture: a breakthrough in vivo. International journal of molecular sciences, 16(3), 5517–5527. doi:10.3390/ijms16035517.
What tools and technologies are needed to fully realize the potential of spheroid culture models?
Debbie King
One of the key parameters for success with spheroid culture is controlling the size of the spheroids. It can be very difficult to get consistent, reproducible results if the starting spheroid culture is not uniform in size and shape. Cell culture tools available on the market now, such as ultra-low attachment plates, facilitate the formation of uniformly sized spheroids for many research applications from low to high throughput modalities.
Hilary Sherman
There always needs to be a little bit of a balance between throughput and complexity in terms of creating models for research. That’s why there are so many options available for 3D research. Low attachment products such as Corning® spheroid microplate and Eplasia® plates are great for creating high throughput 3D models, but can lack some complexity. Organ-on-a-chip and hydrogel models add biological complexity to the model, but are typically not as high throughput.
What is the most interesting achievement so far in using organoids?
Elizabeth Abraham
Personalized medicine. Due to their unique ability of unlimited self-renewal, organoids are different from spheroids. Organoids can be made from patient-derived stem cells in a selective medium containing Corning® Matrigel matrix. These organoids can then be exposed to varying drugs to identify the best treatment to fight that particular cancer; thus personalizing medicine to treat disease. Taking this idea even further is the ability to repair genes in cells that can form organoids, then using those organoids to understand treatment regimens. Organoids thus serve as a converging platform for gene editing, 3D imaging and bio-engineering. Thus the therapeutic potential of organoids in modeling human disease and testing drug candidates is in my opinion the most interesting achievement thus far.
Audrey Bergeron
There has been a recent report of researchers at the Cincinnati Children’s Hospital Medical Center (2) developing the world’s first connected tri-organoid system, the human hepato-biliary-pancreatic (HBP) organoid. This is a remarkable achievement since it moves the field from individual organoid research to connected organoid systems, which more physiologically mimic the interplay between human tissues. There have also been challenges to date with current liver organoid approaches failing to adequately recapitulate bile duct connectivity, which is important for liver development and function. The authors describe optimized methods to create the multi-organ model from differentiated human pluripotent stem cells via the formation of early-stage anterior and posterior gut spheroids which fuse together and develop into hepatic, biliary and pancreatic structures. This is an exciting new basis for more dynamic and integrated in vitro systems-based organoid models to study organogenesis, for use in research and diagnostic applications and for potent applications in precision medicine and transplantation studies.
(2) Hiroyuki Koike, Kentaro Iwasawa, Rie Ouchi, Mari Maezawa, Kirsten Giesbrecht, Norikazu Saiki, Autumn Ferguson, Masaki Kimura, Wendy L. Thompson, James M. Wells, Aaron M. Zorn, Takanori Takebe. Modelling human hepato-biliary-pancreatic organogenesis from the foregut–midgut boundary. Nature, 2019; DOI: 10.1038/s41586-019-1598-0.
Debbie King
The generation of cerebral organoids is one of the most interesting achievements. These “mini-brains” derived from pluripotent stem cells can self-organize into functioning neural structures. Neural cells are notoriously difficult to culture in
vitro and obtaining sufficient cells for experiments can be challenging. Cerebral organoids offer a way to study neural tissues, replicating aspects of human brain development and disease that was once impossible to observe in the laboratory. Scientists have used them to make discoveries about neurological disorders like schizophrenia and autism. These organoids have been useful models to examine fetal brain microcephaly caused by Zika virus infection.
What technologies played have helped scientists overcome the biggest challenges to using organoids?
Elizabeth Abraham
3D extracellular matrix, like Matrigel® matrix, provide the appropriate scaffold to be able to generate and grow organoids. This matrix can also be modulated by matrix metalloproteases secreted by cells within the organoid to grow and differentiate.
The discovery of Wnt signaling is also important as the Wnt pathway is the heart of the organoid technology.
Lastly, 3D imaging, the ability to see inside 3D structures such as organoids has also enabled scientists learn how to use organoids in disease modeling.
Audrey Bergeron
One of the biggest challenges is the lack of vasculature in organoid systems, which hinders in vivo-like expansion and limits organoid size. Technologies have been developed (and are continuing to be developed) which improve long-term culture conditions and the delivery of nutrients and gaseous exchange to the developing organoid. These include spinner flasks and bioreactors to increase “flow” in the culture system and microfluidics-based platforms for efficient nutrient diffusion, oxygenation and waste metabolite disposal (a key example is cerebral organoids).
It is also interesting to see the evolution of permeable membranes such as the Transwell® permeable supports and other semi-permeable membrane materials being integrated into perfusion systems and 3D bioprinting techniques to improve nourishment to the organoid during maturation. These technologies have helped to increase the life- span and utility of organoids to months.
Another challenge in high throughput pharmacological and toxicity screening applications has been the formation of reproducible, single organoids per well. The Ultra-Low Attachment (ULA) surface cultureware or microplates coupled with established biological hydrogels such as Corning Matrigel matrix have provided a platform to generate uniformly sized organoids compatible with HTS applications. Concurrent advancements in high content screening platforms has also helped to elucidate the 3D complexity of organoids in terms of multi-parameter imaging and quantitative analysis.
What advancements do you see with organoids in the next five years?
Elizabeth Abraham
Organoids fulfilling the need of “organ-donors” that can be used in patients awaiting transplantation and using organoids as a diagnostic tool to detect and treat cancers.
Audrey Bergeron
More complex, vascularized multi-organoid systems will continue to be developed to advance precision and regenerative medicine closer towards transplantable organs. I also think that protocols and models will continue to be optimized to generate data and improve clinical predictively of organoid models in pharmacological and toxicity testing – this could potentially mitigate the need for animal models during drug development.
Debbie King
Researchers are also looking to combine genome-editing technologies like CRISPR-Cas9 in particular with patient cell-derived organoids. For monogenic diseases, it opens up the possibility of performing gene correction through gene editing prior to autologous transplantation as a curative solution. It’s already been shown that the defective CTFR gene in cystic fibrosis patient-derived organoids can be corrected using CRISPR/Cas9 homologous recombination (3).
(3) Schwank G, Koo BK, Sasselli V, Dekkers JF, Heo I, Demircan T, Sasaki N, Boymans S, Cuppen E, van der Ent CK, Nieuwenhuis EE, Beekman JM, Clevers H. Functional repair of CFTR by CRISPR/Cas9 in intestinal stem cell organoids of cystic fibrosis patients. Cell Stem Cell. 2013 Dec 5;13(6):653-8. doi: 10.1016/j.stem.2013.11.002.
What technologies will enable those achievements?
Hilary Sherman
I think more defined reagents such as ECM’s and media will help to make organoid culture easier and more consistent. Also, better bioprinters with higher resolution will aid in generating more complex 3D structures.
Debbie King
Automation platforms will allow for precise control of culture conditions and enable high-throughput screening in drug discovery workflows. Also, high-content imaging technology will be key to capturing morphological and gene expression data to study organoids. Live cell imaging within organoids will allow us to visualize, for example, early events in human development in real time. Overall, the field would also benefit from standardization in protocols, reagents such as the type of culture media/ECM to use and the best cell sources so that comparisons between labs can be made to help advance research forward.
What areas of research do you think will be most impacted by 3D culture systems?
Audrey Bergeron
Cancer research, to better model cancer in vitro to better understand cancer biology and for personalized medicine.
Hilary Sherman
Regenerative medicine, a branch of therapy that involves engineering biological tissue or organ to establish normal function, this includes the hope to someday be able to 3D print organs.
Debbie King
3D culture systems will continue to have a large impact on developmental biology. To study human development, this has largely been limited to observational studies on pre-implantation embryos or from progenitor cells isolated from fetal tissues, which are then cultured in vitro. The advent of organoid models derived from iPSCs opens up the ability to study human embryonic development in a way we couldn’t do before. As well, organ-specific progenitors generated from iPSCs provide a wealth of insight into the morphogenesis of different organ systems.