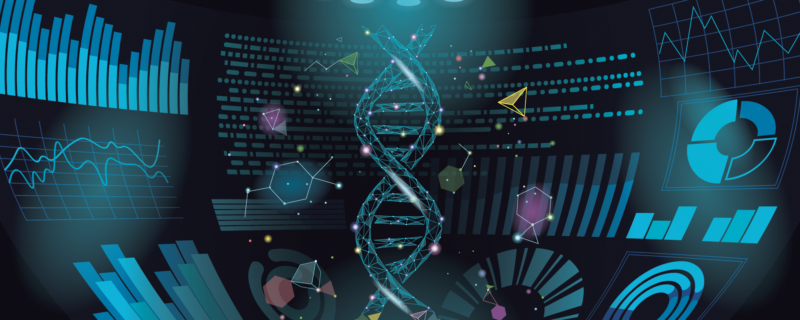
Cell and Gene Therapy Advancements, Challenges, and the Path to Commercial Success
Cell and gene therapies are undeniably one of the most exciting medical innovations of the 21st century with the potential to provide effective medical cures in a variety of disease states previously thought to be incurable or untreatable. While we are still in relatively early stages for these new treatment modalities compared to other biologics like monoclonal antibodies (mAbs), the approvals to-date in rare and ultra-rare disease indications have redefined the boundaries of therapeutic possibility and captured global attention. As of this writing, 14 cell and gene therapies have been approved by the FDA in the United States, twelve highlighted at the annual meeting of American Society of Gene & Cell Therapy (ASGCT)1, with two recent approvals coming in June. Additionally, there are around 2,000 gene therapy products2, including CAR-T cell therapies and other genetically modified cell therapies under development, and more than 3,000 in pre-clinical development3.
In 2023 to date, we have witnessed the approval of the first gene therapy treatment for Duchenne Muscular Dystrophy (DMD), Elevidys™ (delandistrogene moxeparvovec-rokl), an adeno-associated virus vector (AAV)-based gene therapy developed by Sarepta Therapeutics. In addition, BioMarin Pharmaceutical Inc.’s Roctavian™ (valooctogene roxaparvovec-rvox), an AAV-based gene therapy to treat severe hemophilia A also received approved this year. If all goes to plan, 2023 could see the first approval decision for a CRISPR genome editing product leveraging a cell therapy platform4, setting high expectations for things to come. The collective achievements in recent years and record-breaking investments signify a growing recognition of the transformative power of this field, igniting hope for patients suffering from diseases with limited medical alternatives. The industry’s commitment is reflected in ongoing research and advancements that bring us closer to realizing the full potential of these therapies.
Cell Culture Dish, in collaboration with a panel of gene therapy experts, published two e-book publications in 2018 and 2020 that covered all aspects of gene therapy commercialization in depth. As the field continues to broaden and mature, we wanted to provide an updated look—with insight from industry experts—at the current landscape across therapeutic targets, manufacturing processes, and regulatory frameworks and how progress in these areas are propelling cell and gene therapies forward. Although there is huge promise, the field is still young and there are many challenges that persist. Here we explore the obstacles that remain on the path to commercial success and highlight the key opportunities for future advancement and sustainability.
A Look Back at the Advancements, Therapeutic Prospects, and Challenges
Our experts agree that cell and gene therapies hold immense potential as novel treatments for rare and ultra-rare genetic diseases, many with no existing therapeutics or with very limited treatment options. The industry is beginning to show signs of maturation, marked by a noticeable shift toward long-term strategies in product development. The initial wave of approvals played a pivotal role in establishing the baseline for an approved gene therapy and how to commercialize these new modalities. This transition from a theoretical concept to a tangible reality has set a crucial benchmark, providing valuable insights into safety factors and product quality attributes that must be considered as candidates progress through chemistry, manufacturing, and control (CMC) and clinical development.
As we move beyond the proof-of-concept stage, there is a growing understanding of the essential elements that contribute to successful outcomes. Analyzing the clinical data, risk-benefit profiles, and real-life patient outcomes from these initial approvals offers the opportunity to uncover commonalities, patterns, and general principles that can be applied to other diseases. These insights enable us to explore a broader range of clinical targets beyond ultra-rare indications and will contribute to the continued advancement of the field towards more effective and accessible treatments.
Therapeutic Targets
In terms of disease targets, cell and gene therapy will continue to have a significant impact in oncology and rare genetic diseases where unmet medical needs are extremely high. Ongoing breakthroughs in the treatment of solid tumors, will also bring forth both opportunities and challenges. About three-quarters of the roughly 180 diseases targeted by the 700 gene therapy trials now underway are rare diseases or cancers5. The scope of treatments being developed is also broadening beyond a small number of monogenetic diseases to more complex applications including metabolic, neurological, kidney, heart, infectious, and respiratory diseases. In addition to traditional cDNA gene therapy, advancements in gene editing, including the use of CRISPR-Cas9, Zinc Fingers, TALE nucleases, and non-nuclease base editors, are driving progress in in vivo genome modification approaches. These innovations hold the potential for longer-term cures and could alleviate the need for repeated treatments in patients. Groups are also exploring the use of cell and gene therapy modalities to complement and significantly boost the therapeutic effect of established treatments, such as small molecules and traditional antibodies.
Currently, the field is racing towards treatments for more straightforward diseases, such as hemophilia, sickle cell disease, or degenerative eye diseases, with several companies directing their efforts in these areas. Speed to market, and especially, speed to patients is paramount for cell and gene therapy innovators. The sense of urgency is undeniable, but it is important to acknowledge that many of these diseases have very small patient populations, so although these treatments could provide cures, the market likely would not support multiple competitors simultaneously. The stakes are high for companies invested in these modalities, as being late to market could translate into missing the opportunity to treat the majority of eligible patients. Such a scenario can profoundly affect a company’s ability to recoup their R&D investments.
It’s a delicate balancing act, driven by the dual goals of delivering life-changing therapies to patients while ensuring the long-term viability of these innovative treatments in a competitive and resource-constrained environment. To expedite the development of these new modalities, strategic partnerships have emerged as a pivotal strategy. These partnerships, whether through targeted collaborations or mergers and acquisitions (M&A), enable smaller biotech firms to join forces with larger, more established biopharma players5. Such alliances aim to accelerate the development and delivery of these innovative therapies to the patients who need them most.
Challenges Remain
While significant progress has been made across the field, there is room for improvement, particularly in manufacturing processes. Issues including the cost of goods (COGs), process robustness, capacity, and scalability will need to be addressed to fulfill current and future demand for materials. As more cell and gene therapies have gained approval in major markets, regulatory expectations have increased as well, with greater emphasis placed on product purity, performance of production processes, and adherence to regulatory standards. Evolving regulatory expectations are challenging product design, manufacturing, and testing procedures to provide greater product understanding that will lead to improved patient safety and product efficacy.
Another significant challenge lies in the delivery of therapeutic genes to specific tissues or cells to both maximize therapeutic efficacy and minimize off-target side effects. Vector delivery is an active area of focus and ongoing research aims to improve the efficiency, safety, and long-term effectiveness of current vectors, such as adeno-associated viruses (AAVs) and lentiviruses. Improved targeting by exploiting serotype-specific tissue tropism or by modifying their surface proteins to improve cell targeting could make it possible to lower the dosage required per patient while still achieving the desired therapeutic effect. This is especially relevant given the high production costs and relatively low per cell yield of viral vectors.
Breaking Through Manufacturing Hurdles
The primary focus in gene therapy currently revolves around AAV-mediated approaches, which is the area where significant advancements and learning are occurring. At present, expertise and infrastructure is skewed toward transient transfection because they allow the greatest flexibility. However, this method involves costly inputs, such as plasmid DNA and transfection reagents, to generate viral particles encapsulating the therapeutic gene. Unlike mAb production, viral vector manufacturing is not yet standardized across the industry, with biopharma companies using different production systems and downstream processes. Cost, potency, meeting critical quality attribute targets, and overall manufacturing capacity to meet dosage demands continue to be significant challenges in viral vector manufacturing.
Overcoming Cost Hurdles
The field is actively addressing the cost challenges associated with viral vector production through focused efforts in vector design, process optimization and improving cell production systems. These integrated approaches have the potential to yield substantial benefits, with the possibility of achieving 5-10-fold increases in overall viral titers.
As discussed previously, refining vector targeting not only reduces dosage requirements but also minimizes the risk of immunogenicity. For AAV specifically, there are currently eleven identified serotypes that differ in their tropism, allowing for vector delivery to specific tissues or organs. Researchers have further refined the tropism of AAV through pseudotyping where the capsid and genome from different viral serotypes are mixed or through novel hybrid capsid designs where capsids from multiple serotypes are combined to improve vector targeting.
Current first-generation vectors require very high doses per patient (i.e., 1E14 vg/kg) resulting in potential toxicity issues and posing significant manufacturing challenges to satisfy the yield requirements for even early-stage clinical studies. Compared to traditional recombinant biologics, the manufacturing processes of AAV and lentivirus provide very low per cell productivity. According to our experts, there are certain disease indications where improved vector design could reduce dosage requirements by >2 logs that coupled with the basic process enhancements. This could result in an estimated 1000-fold (or more) improvement in doses per batch, significantly reducing clinical development costs and the price tag of commercial products.
While optimization efforts are underway for three-plasmid transient transfection protocols to achieve better yields and scalability, some companies are exploring two-plasmid systems for AAV production. This transition could greatly simplify processes, reduce COGs, and enhance process consistency. Beyond transient transfection, the focus of viral vector research and funding is focused on the accelerated development of high-yield producer cell lines that eliminate the need for costly raw materials and transient transfection to streamline manufacturing processes, reduce costs, and enable greater scalability, and process intensification.
Achieving Process Robustness
To achieve the successful commercialization of cell and gene therapies, robust manufacturing and control systems are crucial to ensure the consistent production of safe, efficacious, and accessible products. Despite claims of “platform processes,” the industry has not yet achieved truly universal and plug-and-play systems that exist for mAbs. The inherent complexity and diversity of cell and gene therapies will require processes to be continuously improved and optimized over time. It is important to recognize the limitations of the current vector production platforms—whether a process is truly platform is still subjective to each developer’s own standards and criteria.
Platform approaches not only reduce the need for extensive process development but help to establish a framework for production documentation and supply chains for key consumables such as single use systems, and test and release procedures to de-risk development. Current non-platform processes require significant process development to establish manufacturing processes required to meet desired attributes outlined in the target product profile (TPP). The relative lack of suitable in-process assays with real-time capabilities to monitor critical parameters like vector titers and purity is cited by our experts as an opportunity for improvement both during the development and production stages.
Establishing industry-wide standards or best practices for manufacturing will require extensive knowledge sharing and consensus from various stakeholders. This may be challenging due to the rapidly evolving nature of gene therapy technologies and the drive to be first-to-market with new therapies. Despite this, industry-wide initiatives and consortiums that facilitate pre-competitive sharing of knowledge, data, and best practices are driving progress in this direction. Additionally, regulatory agencies can promote standardized approaches by encouraging transparency and providing clear guidance on manufacturing processes. It will be necessary to strike the right balance between process standardization for consistency, reliability, and scalability while leaving room for continued innovation and improvements.
Scalability Challenges
Currently, all approved viral vector in vivo therapies are produced in adherent transient-transfection systems, but they quickly reach their limits in terms of scalability6. To increase batch yields, many companies are shifting towards scalable transient-suspension systems. Large volume stirred tank bioreactors in suspension cultures also improve process controls and consistency while managing costs effectively. Adapting adherent cells to suspension culture is time-consuming and feasibility depends on factors like cell line, vector type, and downstream processing considerations. That said, there are several suspension-adapted cell lines for transient transfection available for commercial licensing on the market, providing options that can be seamlessly integrated into viral vector production processes.
Alternative production systems including Sf9 insect cell-based baculovirus expression vector (BEV) and producer cell lines are currently the most scalable and cost-effective6. However, it’s important to note caveats such as the lower infectivity of viral vectors produced in the BEV system because of nonmammalian post-translational modifications that necessitate a higher dosage and the resource-intensive and time-consuming process of generating stable producer cell lines.
It’s clear there is no one-size-fits-all system capable of meeting the requirements of all cell and gene therapy products. Companies must devise a strategy tailored to their specific portfolio, carefully considering resource allocation, risk management, the size of the potential patient population, and the market landscape.
Enhancing Vector Purity
AAV products face challenges related to vector purity, particularly in the separation of full capsids from empty or partial capsids, as well as the efficient removal of host cell contaminants. One common quality indicator is a high ratio of full to empty capsids, as empty or partial capsids can diminish therapeutic outcomes and may trigger unwanted immune responses in patients. There have been advancements around analytical capabilities with the introduction of droplet digital PCR (ddPCR), which is a more precise and absolute quantification method compared to conventional PCR and qPCR. Since ddPCR may also detect partial encapsidated vector, orthogonal methods such as SV-AUC (sedimentation velocity analytical ultracentrifugation) and CD-MS (charge detection mass spectrometry) have become invaluable for characterizing and differentiating full, partial, and empty AAV capsids based on their payload content.
Experts emphasize the importance of both vector design and improved purification techniques to reduce impurities and achieve process consistency. These areas are also receiving heightened regulatory attention to ensure product safety and efficacy.
Implementing Automation in the Digital Era
Manufacturing for cell and gene therapies has relied heavily on manual processes thus far, making production labor-intensive and vulnerable to human error, contamination, and batch variability. Automated technologies including single-use bioreactors with built-in monitoring capabilities, as well as separation and purification systems like chromatography and tangential flow filtration, can remove the need for manual intervention and improve overall consistency, thereby enhancing process control. Another area where automation can have an impact is in early-stage development during the initial screening of transfection conditions and identifying high-producing clones. Miniaturized plate-based assay approaches with automated robotic liquid handlers and plate handlers can increase throughput and accelerate the iterative process.
While there is undoubtedly a strong appetite for incorporating automation, many gene therapies move through pre-clinical and clinical phases in regulatory fast-track programs. And, due to the small patient population, data points are limited, making it difficult to develop and implement effective automation strategies. Equipment manufacturers could be a key part of the solution through the provision of equipment systems that offer agnostic and flexible capabilities. This would empower developers to establish a process-specific platform using the same equipment from process development to product commercialization. These systems utilize open architecture control systems, enabling them to adapt to the required level of automation. As the process matures, the software can be modified through various iterations to achieve full automation with compliance to Batch and 21 CFR regulations or any level of automation in between.
Automation can also support the digitization of the manufacturing processes to enable real-time process monitoring. Equipment systems with built-in Historians that log and display the data in a digestible way can facilitate the development of analytics-based models to achieve process optimization and consistency. By eliminating paper-based batch record systems, automation moves process control, data collection, analysis, and storage electronically, which fosters faster decision-making and rapid release of gene therapies to the clinic.
Looking ahead, automation will be a critical enabler to expand production capabilities to meet the surging demand for cell and gene therapies. The sector is already experiencing the effects of the skills gap, which is only expected to widen in the short term. Consequently, recruiting and training sufficient staff to support the significant expansion of gene therapy production and achieve the necessary cost reductions will be a major challenge and automation could be a key part of the solution.
Addressing the Capacity & Workforce Shortage
The “capacity crunch” across the sector is well-known, with current reports indicating a shortage of 500% in manufacturing capacity7. Substantial investments are being made to build new facilities and expanding existing ones to meet the increasing demand. Many larger biopharmaceutical companies have opted to invest in their own in-house manufacturing capabilities. This move allows them to safeguard proprietary technologies, maintain full control over the manufacturing process, and meet the material demands during clinical development and potential commercialization of their own therapeutic candidates without having to face waitlists at a CDMO that can stretch one to two years.
However, smaller companies need to partner with CDMOs to leverage their manufacturing expertise without significant upfront investments. Since many developers are new companies in a novel space, they heavily rely on their CDMO partners to offer valuable guidance and ensure the successful development and manufacturing of their therapies. With demand for CDMOs at an all-time high, manufacturing startups8 are making a play for market share, so taking the time to choose the right partner can be crucial to success. The right CDMO will possess extensive experience, technical proficiency, and regulatory knowledge across the entire manufacturing process, including development, analytics, CMC, and commercial-scale production.
In addition to the capacity crunch, the industry is grappling with a substantial shortage of trained professionals that is threatening the development of new therapies. A recent Alliance for Regenerative Medicine Workforce Report9 revealed that 73% of subject matter experts surveyed identified quality control, analytical development, and manufacturing as the most challenging positions to fill within the sector. Even the FDA has encountered difficulties in expanding its staffing levels to keep up with the growing demands of regulatory activities.
The driving force of these shortages is a lack of education, and the industry needs to implement strategies to grow and train an entire workforce around the development and production of these products if they are to reach their commercial potential. Currently, much of the manufacturing training happens “on the job” because although some universities and academic institutions offer courses related to bioprocessing and regenerative medicine, the number of training opportunities specifically tailored to gene therapy manufacturing falls short of the industry’s requirements. This is further compounded in a field where technological advances outpace traditional curriculums.
To address this issue, government, academic institutions, and industry stakeholders are collaborating on long-term initiatives to bridge education gaps. The Biden-Harris Administration is taking action to strengthen and diversify the US biotechnology and biomanufacturing workforce10. They have unveiled a comprehensive plan to collaborate with employers, unions, and other stakeholders to expand biomanufacturing education and job training programs at both secondary and postsecondary levels.
Upskilling the current workforce and leveraging manufacturing professionals from related fields, such as monoclonal antibodies (mAbs) or protein production with transferable skills could provide a faster route to a stable and competent workforce in the short term. Digital tools like augmented and virtual reality could also be leveraged to train operators “in place”, whether for initial onboarding or for infrequent processes that require refreshers6. The problem isn’t solvable overnight, but by continuing to pursue short- and long-term solutions and making strategic investment in workforce development, the industry can work to bridge the skills gap to ensure long-term sustainability.
The Current Regulatory Landscape
Regulatory agencies are ensuring their readiness to meet the growing pipeline of cell and gene therapies and looming boom in Investigational New Drug (IND) submissions. The FDA has established a new Office of Therapeutic Products (OTP) in March 2023 at the Center for Biologics Evaluation and Research (CBER)3. The super office includes six sub-offices, 14 divisions, and 33 branches, focused on streamlining workflows and improving efficiencies within the agency.
Despite their readiness, the FDA acknowledges that the current regulatory framework for cell and gene therapies needs updating to better address the unique challenges introduced by these products. The fast-track regulatory pathways implemented by the FDA such as Breakthrough Therapy Designation, Regenerative Medicine Advanced Therapy (RMAT) Designation, and Priority Review, demonstrate the agency’s willingness to collaborate with companies and expedite the approval of novel gene therapy products. Additionally, effort has been made to classify advanced therapies into subclasses such as “n of 1’s”, ultra-rare, and rare diseases. These subclasses are pushing the industry and FDA alike to consider how best to demonstrate process consistency and approve a product with a very limited number of batches, especially for n of 1 and ultra-rare products. Traditional approaches to Chemistry, Manufacturing, and Controls (CMC) may not always be applicable in these cases.
One area of confusion is the need to maintain GMP (Good Manufacturing Practice) quality requirements for starting materials like plasmids, for which there are no specific regulations in the Code of Federal Regulations (CFR). Obtaining the necessary GMP information from starting material manufacturers, as required by regulators, can be difficult. To address this issue, many companies or CDMOs have opted to bring plasmid manufacturing capabilities in-house. Plasmid manufacturing relies on microbial fermentation, presenting challenges to ensure proper segregation from mammalian cell culture processes.
In September 2022, the FDA addressed GMP requirements for plasmids during an FDA OTAT Town Hall11 [see 42:54 – 46:23 min]. The FDA stated, “US regulations do not require that plasmid starting material be made under strict GMPs…, yet goes on to say, “plasmid quality is critical for drug product manufacturing.” The use of research-grade plasmids in advanced therapies for Phase 1 clinical trials can present challenges when these plasmids do not fully meet GMP quality requirements necessary for later-phase trials and commercialization. The FDA’s Phase 1 drug product (DP) GMPs has been transformative because they define the minimum GMPs that must be applied for Phase 1 / 2 clinical trial material. A similar guidance for early-phase critical raw materials is needed to avoid hindering the introduction of breakthrough advanced therapies while still ensuring their safety and efficacy during clinical trials.
Ensuring Long-term Success: Business Sustainability and Affordability of Cell and Gene Therapies
There are growing concerns about the commercial viability and financial sustainability of these transformative treatments. With only a small number of licensed products, some of which carry million-dollar price tags per treatment, cell and gene therapies diverge from the traditional drug model by offering one-time treatments with curative potential. While advantageous for patients, this unique characteristic raises questions about the revenue stream for biopharma companies and their ability to fund ongoing research and development. The commercial viability is a key factor right from the early stages of product development, where justifications must be made about profitability.
Many genetic disorders are rare with small patient populations. Others are rarer still, where these “n of 1” (bespoke) diseases affect only a few dozen (or fewer) individuals. While these therapies are vital for patients with these conditions, the limited market size can make it challenging for companies to recoup their investments. The prospect of being able to price treatments in the millions of dollars might not be enough if companies need to spend much more to collect the required clinical evidence and, in the end, can only treat a few patients. We’ve witnessed this first-hand with gene therapy treatments for several types of severe combined immunodeficiency (SCID) that could be curative. Despite this, companies have backed off, deprioritizing these programs because of concerns over commercial viability and uncertainty about achieving returns like more mainstream therapies.
Obtaining reimbursement from healthcare payers is another hurdle. Negotiating pricing and demonstrating long-term value can be complex. In response, companies are exploring innovative pricing models, value-based agreements with payers, and partnerships or innovative funding models may help spread the financial burden and make cell and gene therapies more accessible to those in need. Finding the right balance between affordability and incentivizing continued research and development will be necessary to continue to propel advancement in the field.
Optimism for the Future of Cell and Gene Therapy
In the next 5-10 years, the cell and gene therapy field is poised for further progress, driven by improvements in delivery systems, vector productivity and quality, gene editing technologies, and a deeper understanding of disease mechanisms. However, to fully unlock the potential of gene therapies, innovation in several areas is still needed. Enhancing vector designs to mask vector immunogenicity, uncovering new (and better) delivery mechanisms, and exploring co-therapies could enable targeting disease areas that are currently out of reach.
Additionally, a significant expansion of clinical and diagnostic capabilities will be essential. These advancements will support ongoing clinical trials and facilitate early diagnosis and intervention for patients with licensed cell and gene therapy products. The success of many these advanced therapies depends on timely and accurate diagnoses, making the development of robust diagnostic tools critical to maximizing the impact of these transformative treatments.
Despite the challenges and complexities surrounding these new modalities, there is a profound sense of optimism and excitement from our experts. The remarkable advancements made in recent years, coupled with ongoing research and innovation, have laid a strong foundation for the continued growth and success of cell and gene therapies. The road ahead may still have obstacles, but the collective determination to overcome them and the shared vision of transforming the future of medicine continue to motivate forward progress.
Footnotes
-
1. Bayer M. Fierce Biotech. ‘Like salmon swimming upstream’: FDA’s Peter Marks lays out plan to boost gene therapy approvals. Fierce Biotech. Published April 5, 2023. Accessed July 24, 2023. https://www.fiercebiotech.com/biotech/thats-failure-fdas-marks-says-gene-therapy-approvals-must-rapidly-increase
-
2. American Society of Gene and Cell Therapy. Gene, Cell & RNA Therapy Landscape Report: Q2 2023. Accessed July 24, 2023. https://asgct.org/publications/landscape-report
-
3. Mulero A. FDA Braces for Looming Boom in Cell and Gene Therapy Submissions. BioSpace. Published June 12, 2023. Accessed July 24, 2023. https://www.biospace.com/article/fda-braces-for-looming-boom-in-cell-and-gene-therapy-submissions/
-
4. Zamecnik A. CRISPR gene therapies: Is 2023 a milestone year in the making? Pharmaceutical Technology. Published January 3, 2023. Accessed July 24, 2023. https://www.pharmaceutical-technology.com/features/crispr-gene-therapies-is-2023-a-milestone-year-in-the-making/
-
5. Fraterman S, Xie W, Wu C, Thielmann M, and Yudhistiara B. New Drug Modalities Offer Promise and Peril. Boston Consulting Group. Published March 1, 2023. Accessed July 24, 2023. https://www.bcg.com/en-ca/publications/2023/benefits-and-risks-of-new-drug-modalities
-
6. Capra E, Gennari A, Loche A, and Temps C. Viral-vector therapies at scale: Today’s challenges and future opportunities. McKinsley & Company. Published March 29, 2022. Accessed July 24, 2023. https://www.mckinsey.com/industries/life-sciences/our-insights/viral-vector-therapies-at-scale-todays-challenges-and-future-opportunities
-
7. Cohen J. Cell And Gene Therapies Face Persistent Manufacturing Capacity Constraints. Forbes. Published July 6, 2023. Accessed July 24, 2023. https://www.forbes.com/sites/joshuacohen/2023/07/06/cell-and-gene-therapies-face-persistent-manufacturing-capacity-constraints/?sh=1c76be92799e
-
8. Wu G. Cell and gene therapy manufacturing: the next generation of startups. BioPharma Dive. Published June 20, 2023. Accessed July 24, 2023. https://www.biopharmadive.com/news/cell-gene-therapy-manufacturing-cdmo-biotech-startups/653275/
-
9. Alliance for Regenerative Medicine. Workforce Report: Gap Analysis for the Cell and Gene Therapy Sector. Published March 15, 2023. Accessed July 24, 2023. https://alliancerm.org/sector-report/workforce/
-
10. The White House. FACT SHEET: Biden-Harris Administration Announces New Action Plan to Bolster, Expand, and Diversify America’s Biotechnology and Biomanufacturing Workforce. Published June 27, 2023. Accessed July 24, 2023. https://www.whitehouse.gov/ostp/news-updates/2023/06/27/fact-sheet-biden-harris-administration-announces-new-action-plan-to-bolster-expand-and-diversify-americas-biotechnology-and-biomanufacturing-workforce/
-
11. Gavin D. FDA CBER OTAT: Gene Therapy Chemistry, Manufacturing and Control Questions & Answers. Online presentation; September 2022. Accessed July 24, 2023. https://fda.zoomgov.com/rec/play/K9Tk9g5XMbFG2D9BbLv4MZtN0QBewz_EnB1iSaA6ZPr_PlRbN6sV_irPlP5w6PqduCWMRkpKxiGqeNZf.iGZkgRjzq8VofTTz?startTime=1664465377000