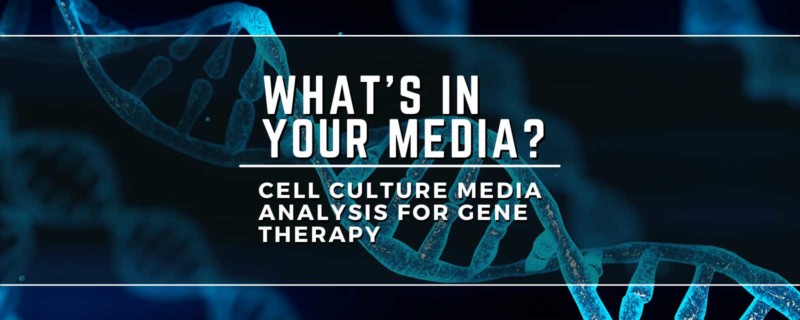
Cell Culture Media Analysis for Gene Therapy
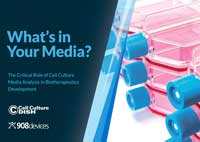
“What’s In Your Media”.
You can download all the articles in the series, by downloading the eBook.
The genes within our cells contain the necessary information to direct the production of proteins and enzymes to support normal functions in the body. However, when a gene or part of a gene is defective, mutated or missing, this can disrupt normal functions manifesting in a number of health problems and diseases. Gene therapy aims to treat diseases through genetic modification, most frequently genetically engineered viral vectors are used to deliver a genetic payload to the cells. There are non-viral methods in development, however, viral vectors are still the most popular approach with two-thirds of the clinical trials to date delivered via viral vector.1 It has the potential to transform medicine and create new therapeutic options for patients who are living with difficult, and even incurable, diseases. The gene therapy can be delivered directly in vivo to affected cells through either providing the cell with working copies of the gene or by silencing mutated genes that function improperly and cause disease. Another gene therapy strategy aims to reengineer cells ex vivo to increase their therapeutic efficacy against a target population. This is the principle behind CAR T-cell therapy where the patient’s own immune cells are collected and modified with viral vectors in a laboratory before being reintroduced to the patient where they can exert their effect against the target cancer cells in vivo.
Delivering and expressing a gene of interest typically relies on viral vector delivery systems derived from lentivirus (LV), adenovirus (AV) or adeno-associated virus (AVV). Of these, AAVs have become the vector of choice for in vivo gene therapies owing to their safety profile, high gene transfer efficiency, stable long-term expression, and selective tissue tropism. The most common way of producing AAV for gene therapy involves transient transfection of three different plasmids into the cells – the transfer plasmid containing the gene of interest, the second with the rep-cap sequences for viral replication and capsid assembly, and the final helper plasmid contains the adenovirus helper functions.
The majority of approved gene therapies are targeted to rare diseases with niche patient populations but the hope is to design targeted therapies for more prevalent disease indications that affect larger patient populations. The increased investment and innovation in the gene therapy space holds great promise for patients but brings with it challenges for manufacturers to meet the high clinical and commercial demand. cGMP plasmid DNA is an essential starting material for viral vector production but current manufacturing strategies based on methods adapted from the basic research laboratory lack the efficiency and consistency to meet yield requirements. These same issues extend to viral vector manufacturers, resulting in high cost of goods (COGS) and bottlenecks along the entire supply chain for gene therapies. Manufacturers are working actively to simultaneously scale up manufacturing processes and increase host cell productivity aimed at addressing these challenges.
Plasmid DNA
As more cell and gene therapies advance to FDA approval, it is essential to scale up current production processes to increase plasmid DNA productivity economically, and at the scale needed to meet industry demand. Plasmid production is commonly achieved through recombinant Escherichia coli fed batch fermentation, during which the appropriate genetic sequences are amplified, harvested, purified, and tested for safety. There can be issues with lot-to-lot consistency in the fermentation media which contains hydrolysates, which in turn imparts lot-to-lot variability in plasmid yield and purity. Added to this is the desire in the industry for costly GMP-compliant manufacturing since plasmid DNA is an upstream raw material for viral vectors in clinical applications, which has imposed increasing levels of GMP stringency where more defined cell culture media/growth conditions without animal-derived components, better in-process monitoring and overall process control are required.2
Typical fermentation media is composed of undefined components, like peptone or hydrolysate mixtures with a wide range of amino acid concentrations (Figure 1) resulting in yield, quality and purity inconsistencies in manufacturing. Here, the REBEL analyzer can be leveraged to check media conformity across different lots of fermentation media and between vendors to mitigate inconsistencies during manufacturing.
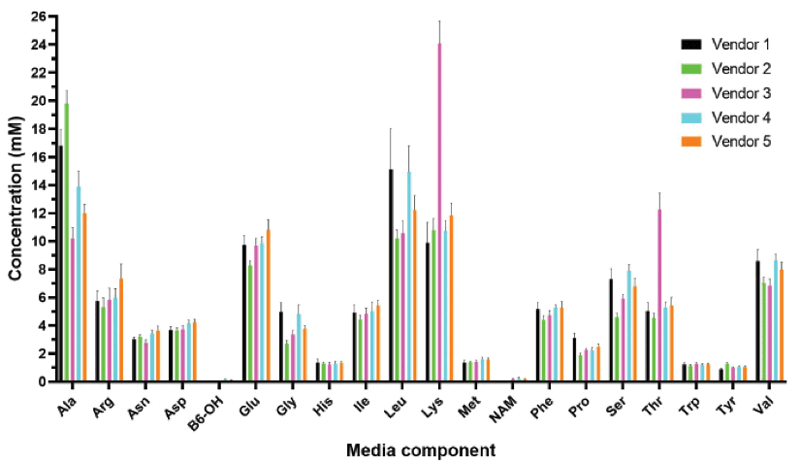
Additionally, in-depth media analysis can aid in the movement towards chemically defined fermentation media to meet increased regulatory standards and to achieve optimal productivity and plasmid quality necessary for downstream viral vector production.
Viral Vectors
Efficient viral vector production is a key element for gene therapy manufacturing where improving overall yield of functional full capsids containing the desired genetic payload is still the biggest hurdle. Typical workflows begin upstream where adherent HEK293 cells, cultured in serum-containing medium, undergo transient transfection with plasmids encoding the main building blocks of the desired viral vector. Viral particles are produced by the HEK293 cells, harvested and put into downstream purification processes. Broadly speaking, one of the main challenges in meeting the high titers required by the industry is how to effectively move from small to large scale commercial manufacture in a reliable and reproducible manner while still maintaining critical quality attributes (CQAs). The complexity of viral vectors —significantly more complex than recombinant proteins, has posed biomanufacturing challenges both upstream and downstream including scalability, productivity, and overall lack of robustness.3 To develop long-term solutions to these problems, manufacturers are looking to optimize both the producer cells and the culture media they are grown in and the mode of culture (adherent vs suspension, fed-batch vs perfusion).14
Scalability
In order to meet market demands in gene therapies, there is a need to improve production efficiencies, which will reduce manufacturing costs and improve patient access. As an example, a phase 1/2 trial for hemophilia B required over 400 ten-layer cell stacks to generate sufficient material for six patients2. While the trial was a success, this study highlights the need for new methods to improve vector generation especially if gene therapies are adopted for larger disease indications.
The ability to culture the production cells at higher densities than is possible in adherent monolayers is one way to increase titers. Adapting adherent HEK293 cells to grow in suspension in a bioreactor system provides a more robust and scalable process enabling higher cell densities to increase productivity as well as reducing overall labor and materials costs.3 However, adapting a cell line to grow in suspension requires medium and process development time that the industry can ill-afford. As an alternative, microcarrier technology for adherent cell lines has also been considered for scale up. This offers advantages of providing a larger surface area for cell growth and the ability to utilize bioreactor platforms that are more suited for large-scale production without the need to adapt the cells to non-adherent conditions.4 In stark contrast to conventional bench-scale static cultures, large-scale suspension bioreactor cultures impart complex hydrodynamic forces on cells due to the fluid agitation required to aid in the transport of nutrients and gasses within the culture volume.5,6 The environmental perturbations and increased cell densities that the cells experience in bioreactor environments may alter their nutrient requirements or require additional additives to serve as a protectant against shear stress7 (i.e., Pluronic F68, methylcellulose or dextran) and thus may require the optimization of the cell culture media in process development.
The use of any animal derived product in medium, including serum, increases the risk of contamination, supply chain instability and variability. However, removing serum and optimizing media for production of viral vectors does require extensive media development and additional time and resource commitment. HEK 293 has been used extensively for transient protein expression, and thus, commercially available chemically defined media for HEK293 may not be optimized for production of viral vectors. Viral vector production is more complex than single protein production, such as monoclonal antibodies. In addition, transfection media may be optimized for high growth, but not for producing high viral titers, therefore significant optimization is required to meet the specific needs of viral vector production culture. Understanding cell metabolism and the impact of medium components, like amino acids and glucose, on titer levels can be done using media analysis studies and is key to increasing productivity.
Additionally, the FDA has expressed concern over the use of HEK293T cells owing to the inclusion of the SV40 large T antigen DNA sequence. This oncogenic construct could pose the risk of tumor development in patients, which has prompted manufacturers to investigate other HEK293 clones and other cell platforms. The baculovirus- Spodoptera frugiperda insect cell (Sf9) platform is an attractive alternative to mammalian HEK293T cells and holds potential as a GMP-compatible viral production system.8 In implementing insect cell lines, the selection of the appropriate media, in particular, the amino acid content, is important because many amino acids required for their growth and/or protein production are not synthesized by insect cells, and must be supplied by the culture media. As shown in Figure 2, the amino acid profile across several commercial formulations of insect cell media varies across a wide range. This global analysis of amino acid content coupled with an understanding of insect cell metabolism can inform media optimization efforts during process development to modulate critical parameters like cell growth/viability and productivity.
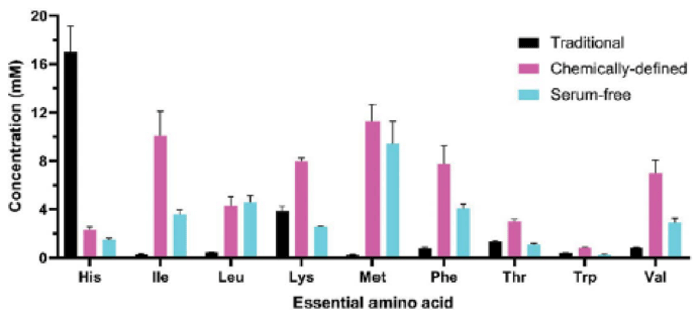
Productivity
The biggest hurdle in viral vector production is yield, not only to increase overall viral titer, but also a need to maximize the full capsids that contains the gene of interest as opposed to partial or empty capsids. Simply put, the more empty capsids there are, the less overall yield. However, the impact of cell culture medium on empty vs. full capsid production is not well defined or understood.
Particularly with AAV vectors, there is a propensity to package any available DNA sequence, which results in capsids that contain illegitimate DNA sequences from the host cell DNA and plasmid DNA or fragmented pieces of the gene of interest. Grimm and colleagues9 first reported that only a small percentage (1.7–20%) of the AAV particles generated in cell culture by transient transfection actually contain vector genomes, the majority corresponding to empty capsids. While we have made significant progress in our understanding of viral capsids as vector technology becomes more mature, the impact of different manufacturing processes on capsid processing, such as post-translational (PTM) modifications are still evolving. Identification of molecular features required for efficient packaging, and modification of production plasmids and the specific culture conditions that can increase the proportion of full capsids can help enhance productivity as well10. The inefficiencies in viral vector production and the noticeable productivity gap compared to other recombinant proteins like monoclonal antibodies is that bioproduction processes for these mature therapeutics have been subjected to years of intense cell culture process development and optimization to define improved media, nutrients, and conditions10. Similar efforts using these approaches that have been effective for other biotherapeutics may substantially improve AAV specific productivity in cell culture.
Safety
As with cell therapy manufacturing, there is a movement towards more defined, animal-free and/or chemically-defined medium that mitigates any potential risk of transfer of viruses or prions into the final product that could induce an undesirable immunogenic reaction in the patient. In addition, it is important to consider whether the vector bulk can be sterilized. For instance, viral clearance is used to some degree in both AAV and Lentivirus processes, which confers a level of viral safety.9 Furthermore, as viral vector demand climbs with more products reaching late-stage large-scale manufacture, supply limitations of certified serum are a real possibility9. In turn, the reduced reliance on serum, the improved lot-to-lot consistency, and the need for more defined mammalian cell growth conditions have driven the development of chemically defined media formulations that are both serum-free and protein-free. Commercially sourced chemically defined formulations are available but many of these formulations are proprietary and thus pose hurdles in optimization for a specific vector product because the components and their concentrations are unknown12, which is where the REBEL can provide valuable intel and insight to drive optimization (Figure 3).
As in monoclonal antibodies where the cell culture medium can also impact PTM and other CQAs, the medium also could have an impact on the capsid PTM and associated CQAs as capsid proteins are glycosylated.13 Also medium optimization needs to be taken into consideration during scale up as cell nutrient requirements can change as the scale of the bioreactor increases.
When developing a new AAV or LV process, decisions have to be made on cell line, mode of culture, and which cell culture medium and feeding strategy to use for optimal productivity and desired CQAs. One common strategy is to perform a screening of commercially available cell culture media. For example there are multiple commercial media available for HEK 293 cells, one of the most used cell lines in viral vector production. As shown in Figure 3, these media can have similar or widely different compositions in terms of amino acids. Is it beneficial to screen as diverse set of medium as possible to maximize the chance to identify a medium that provide the right nutrients to a specific HEK293 cell line. There are many sub-types of HEK 293 also available and each one may need a different balance of AA and vitamins.
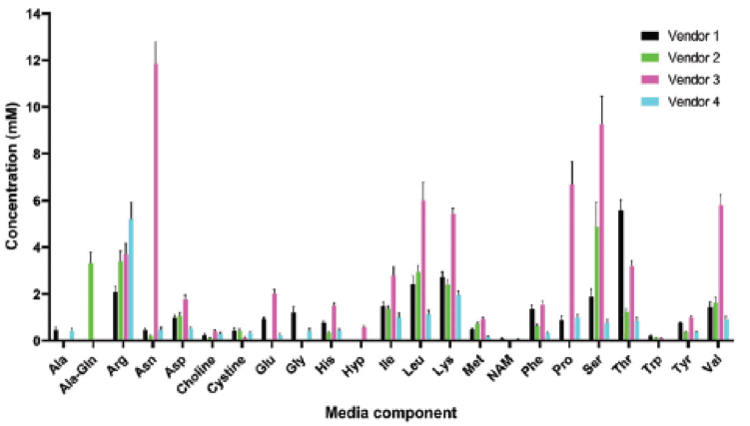
Culture Media Characterization & Optimization
It is evident throughout these discussions that there are many opportunities to improve manufacturing efficiencies for both plasmid DNA and the viral vectors where the cell culture media used for the production cell lines is vitally linked to their productivity and is a critically important aspect of any manufacturing platform. In-depth characterization could aid in optimization efforts to decrease COGS and improve the productivity of host cell lines through better understanding of nutrient requirements or to facilitate process development efforts such as adapting adherent cells to suspension culture and more. Ideally, the media selected should provide the essential nutrients to facilitate high productivity, consistency and quality as well as being amenable to support scale up to larger manufacturing platforms.
At-line and frequent spent media analysis can enable a faster in-house process development pace since there is no need to wait for samples to be processed at a core lab or through outsourced contract services especially with the BSL2 safety requirements associated with viral vectors for transport and handling. The small footprint of the REBEL analyzer allows it to reside in the process development space or manufacturing suite alongside the bioreactors. Leveraging CE-MS technology, the REBEL can produce a quantitative list of amino acids, dipeptides, water soluble vitamins and amines in under ten minutes with very small sample volumes that can facilitate near the real-time decision making necessary to drive forward progress in a rapidly advancing industry.
Footnotes
-
1. Ginn, S. L., Amaya, A. K., Alexander, I. E., Edelstein, M. & Abedi, M. R. Gene therapy clinical trials worldwide to 2017: An update. J Gene Med 20, 3015 (2018).
-
2. Hitchcock T. Manufacturing Plasmid DNA: Ensuring Adequate Supplies for Gene and Cell Therapies. Bioprocess International. October 17, 2016. https://bioprocessintl.com/manufacturing/cell-therapies/manufacturing-plasmid-dna-ensuring-adequate-supplies-gene-cell-therapies/.
-
3. Mirasol F. Modernizing Bioprocessing for Gene Therapy Viral Vectors. Pharmaceutical Technology 2020;44(10):28–33
-
4. Zhao H, Lee KJ, Daris M, et al. Creation of a High-Yield AAV Vector Production Platform in Suspension Cells Using a Design-of-Experiment Approach. Mol Ther Methods Clin Dev. 2020;18:312-320. Published 2020 Jun 3. doi:10.1016/j.omtm.2020.06.004
-
5. Yang J, Guertin P, Jia G, Lv Z, Yang H, Ju D. Large-scale microcarrier culture of HEK293T cells and Vero cells in single-use bioreactors. AMB Express. 2019;9(1):70. Published 2019 May 24. doi:10.1186/s13568-019-0794-5
-
6. Weidner T, Druzinec D, Mühlmann M, Buchholz R, Czermak P. The components of shear stress affecting insect cells used with the baculovirus expression vector system. Z Naturforsch C J Biosci. 2017;72(9-10):429-439. doi:10.1515/znc-2017-0066
-
7. Berry JD, Liovic P, Šutalo ID, Stewart RL, Glattauer V, Meagher L. Characterisation of stresses on microcarriers in a stirred bioreactor. Applied Mathematical Modelling. 2016;40(15-16): 6787-6804 doi: 10.1016/j.apm.2016.02.025.
-
8. Merten OW. Advances in cell culture: anchorage dependence. Philos Trans R Soc Lond B Biol Sci. 2015;370(1661):20140040. doi:10.1098/rstb.2014.0040
-
9. Petit S, Glover C, Hitchcock T, Legmann R, Startt D. Upstream Manufacturing of Gene Therapy Viral Vectors. Cell Culture Dish. January 20, 2021. https://cellculturedish.com/upstream-manufacturing-gene-therapy-viral-vectors-2/.
-
10. Grimm D, Kern A, Pawlita M, Ferrari F, Samulski R, Kleinschmidt J. Titration of AAV-2 particles via a novel capsid ELISA: packaging of genomes can limit production of recombinant AAV-2. Gene Ther. 1999;6(7):1322-1330. doi:10.1038/sj.gt.3300946
-
11. Wright JF. Transient transfection methods for clinical adeno-associated viral vector production. Hum Gene Ther. 2009;20(7):698-706. doi:10.1089/hum.2009.064
-
12. Gélinas JF, Davies LA, Gill DR, Hyde SC. Assessment of selected media supplements to improve F/HN lentiviral vector production yields [published correction appears in Sci Rep. 2018 Apr 25;8(1):6774]. Sci Rep. 2017;7(1):10198. Published 2017 Aug 31. doi:10.1038/s41598-017-07893-3
-
13. Aloor A, Zhang J, Gashash EA, Parameswaran A, Chrzanowski M, Ma C, Diao Y, Wang PG, Xiao W. Site-Specific N-Glycosylation on the AAV8 Capsid Protein. Viruses. 2018 Nov 17;10(11):644. doi: 10.3390/v10110644. PMID: 30453606; PMCID: PMC6266768.
-
14. Vaccine. 2015 Nov 4;33(44):5974-81. doi: 10.1016/j.vaccine.2015.05.097. Epub 2015 Jun 11. Influence of HEK293 metabolism on the production of viral vectors and vaccine Emma Petiot 1, Miroslava Cuperlovic-Culf 2, Chun Fang Shen 2, Amine Kamen 3