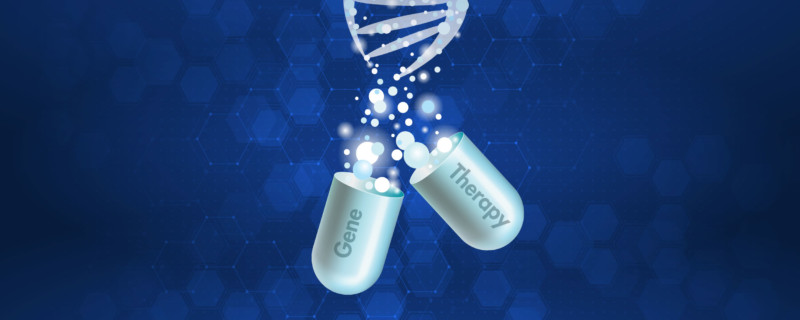
Key Considerations for Gene Therapy Commercialization
Gene Therapy Industry
Gene therapy is the use of a gene-modifying technology to repair, replace or correct damage in the body. The first approved gene therapy study was conducted by the National Institutes of Health (NIH) in 1989 and provided evidence for the first time that human cells could be genetically modified and returned to the patient without harm. To date, approximately 2,600 clinical trials and six gene therapy products have been approved in various countries.1
While the majority of current trials are still in Phase I or Phase II (combined ~ 77%), there are over 100 trials worldwide that are in Phase III or IV. This suggests there could very well be dozens of gene therapy product approvals in the coming years. Last year was a significant year for gene therapy with two pioneering Chimeric Antigen Receptor T Cell (CAR T) cell therapies for cancer approved in the US: Novartis’ Kymriah® and Kite/Gilead’s Yescarta®. Additionally, in late 2017, Spark’s Luxturna® became available to correct a retinal disease.
In light of the impressive remission rates and the recent regulatory approvals, it is no surprise that the gene therapy market has also increased significantly with $7.5 billion raised in 2017 by advanced cell and gene therapy companies.2 Cell and gene therapies have been called the future of medicine and stand to revolutionize the current healthcare paradigm from patient treatment to drug supply logistics.
How Does Gene Therapy Work?
For gene therapies to work, genetic material must be introduced into cells to treat disease, which is most effectively achieved using a vector delivery system. Viruses make good vectors for delivering genetic material because they have evolved to do just that—deliver genes by infecting cells. Viral vectors for gene therapy are modified to ensure that they don’t cause infectious disease in the patient. The most commonly used viral vectors for gene therapy include retrovirus, adenovirus, adeno-associated virus (AAV), and lentivirus. While non-viral approaches for delivering gene therapy are being explored, viral vectors are still the most popular approach with two-thirds of the clinical trials to date delivered via viral vector.1
CAR T cell-based gene therapies have been able to provide unprecedented remission rates and have demonstrated success where other therapies have failed.
Gene therapies can be delivered by injecting the vector directly into the patient (in vivo) or the vector can be delivered to specific cells harvested from a patient’s blood or tissue collection (ex vivo). The vector is introduced into the patient’s cells using a method called transduction. With ex vivo approaches, the modified cells are subsequently expanded in cell culture before they are injected back into the patient.
Non-viral approaches, offer some advantages including delivery of larger genes, simplified production, and reduced biosafety concerns. However, they have been shown to be less efficient at delivering the genetic material, and in some instances, the therapeutic benefits have been short term. Recent improvements in non-viral methodologies are increasing interest in this approach.
Indications
Perhaps the most talked about gene therapies have been the cell-based gene therapies for cancer immunotherapy called CAR-T. Novartis’ Kymriah® and Kite/Gilead’s Yescarta® are examples of these types of cell-based gene therapies. These cell-based gene therapies have been able to provide unprecedented remission rates and have demonstrated success where other therapies have failed. Cancer is by far the largest category of indications being investigated with 65% of the gene therapy clinical trials in this area. The second most popular category of indications is inherited monogenetic disease with 11.1%, followed by infectious diseases (7%) and cardiovascular diseases (6.9%) rounding out the top four indications.1
Keys to Successful Gene Therapy Commercialization
With clinical success and increased investment from the market, many gene therapy companies are looking toward manufacturing and commercialization of their lead therapies. As gene therapy commercialization is relatively new—only six approved products worldwide—there are still several challenges that must be considered when looking at how these products will be manufactured consistently, at an appropriate scale, and delivered to patients. Complicating this task is the fact that until recently there hasn’t been much in the way of enabling manufacturing technologies designed solely with gene therapy manufacturing in mind. Additionally, there is no “one size fits all” approach as gene therapy products are complex and can be manufactured in a variety of ways, using a variety of vectors and cell lines. Lastly, in the case of ex vivo therapies where genetic modifications are made to harvested patient cells, they can also include a cell culture component. These autologous therapies using the patient’s own cells, adds an additional layer of complexity to manufacturing. The logistics of collection and delivery of cells to and from patients can be challenging and often requires a tight turnaround time. And of course, all this must be achieved at the lowest possible cost while still maintaining stringent quality standards to ensure patient safety and comply with regulatory guidelines.
Gene therapy companies should also evaluate whether their current Intellectual Property (IP) portfolio offers them sufficient freedom to operate in terms of commercial manufacturing. The IP landscape around the critical components of the process, including unit operations, needs to be understood and evaluated as soon as possible. Developers can then identify barriers and find alternatives early on in the development process. This will save time and money for the developer.
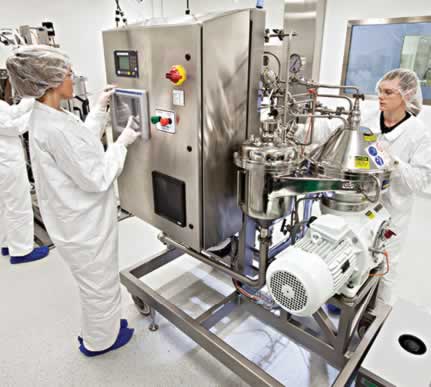
It sounds daunting, but there are excellent resources available and thanks to the 2017 approvals, a recent and successful regulatory and manufacturing pathway has been paved for other companies to follow. In this article, we have pooled many years of experience with experts in various areas of gene therapy manufacturing to create a list of key considerations and best practices.
Product Efficacy and Safety
For therapeutic products to be made available for the general public, products must demonstrate efficacy and safety. Considering the biology of gene therapies, durable efficacy and long-term safety are required by regulatory agencies. Data demonstrating durable efficacy will help to persuade payers and providers that these potentially higher priced therapies are better options for patients compared to traditional therapies. Payers will likely want to see data beyond clinical trials to justify long-term product coverage.
For gene therapies that provide a modest improvement over standard of care, payers will expect the price to be commensurate to the level of improvement. For gene therapies that provide transformative improvement over standard of care, for example a cure, payers will want to look at long-term data with a larger group of patients to justify the higher upfront cost and overall economic benefit of these medicines.
Gene Therapy Manufacturing
Gene therapy manufacturing is a critical part of whether a gene therapy will be successfully commercialized or not. Can the product be manufactured in the quantity and of the quality needed to meet demand? Can it be manufactured at a cost that makes it accessible for patients? These are just some of the issues that need to be addressed.
It is important for stakeholders to include process development and manufacturing programs as early as possible in development to keep pace with the rapid movement of gene-based products through the clinical landscape. There is a key balance to be struck between investing too early in manufacturing technology before the product has been fully characterized and running the risk that the manufacturing process doesn’t produce the correct product. On the opposite end, investing too late means attempting to scale up a process that may not meet needs, which could become very expensive and risky.
Gene therapy companies also need to understand what regulatory pathways are available to them based on whether their product fills an unmet need or addresses a serious, life-threatening condition. As such, there are several expedited pathways that may be available for gene therapy developers. If the product is designated under an expedited timeline then this will impact manufacturing timelines and thus needs to be considered during process design, scale-up, qualification, and continuous verification.
Viral vector systems are by far the most widely used methods to delivery therapeutic gene products because of their infectious nature and ability to introduce specific genes into a cell. Most often, the therapeutic DNA is delivered using viral vector systems based on adeno associated viruses (AAV), lentiviruses (LV), and adenoviruses (Ad). Of these, AAV-based vectors are the most widely used vehicles for delivery in gene therapy indications. According to market experts, AAVs are used in nearly 50% of the 483 currently ongoing gene therapy trials.3 However, a major hurdle for translation of promising research through clinical evaluation to market approval of these therapeutics is meeting the demand for large quantities of viral vectors that this requires. The vector production gap is one of the main challenges facing the industry today. Key elements to the development of large-scale, optimized production are harvesting and purification strategies and the analytical tools to monitor quality attributes ensuring a safe and efficacious product. For the purposes of this article we will focus on viral vector manufacturing.
Key Factors to be Considered in Designing the Manufacturing Process
Evaluating the Existing Process
Many pre-clinical processes for making viruses are currently based on academic protocols where scale and quality are not of the highest importance. It is important to determine whether the current process is scalable to clinical and commercial manufacturing and whether quality demands can be met. Gene therapy developers can have a wide range of perceptions as to how mature their manufacturing processes actually are. The Good Manufacturing Practices (GMP) requirements for raw materials, cell substrates and process consumables are much more demanding and the potential long lead times for obtaining those critical items can be challenging. Therefore, evaluating existing processes will help design process development for clinical and commercial manufacturing. It will also help to develop accurate time lines as academic processes that need more changes will take longer to move to a clinical/commercially compatible process.
Scalability
Scale is one of the biggest considerations when designing the manufacturing process. This can be a challenge to achieve, particularly if a product’s approval timeline is accelerated. Determining scale means identifying how much material is needed for clinical trials and ultimately commercial manufacturing. Projected manufacturing scale is calculated by taking the number of patients to be treated per year x dose / desired number of batches per year.
So for example, if you plan to treat 200 patients per year at a dose of 1014 viral genomes (vg) per patient, then one needs to understand how much a process and a facility can make in a single batch. For example, if a facility has a process that generates 2 x 1015 vg per batch, then the program will need upwards of 10 GMP batches produced, tested and released per year. Based on assumptions about upstream and downstream yields, the scale of the process can be determined. This calculation gets refined through the course of process development and different clinical phases as real numbers get substituted for the assumptions that are initially made. For instance, a 100% success rate is highly unlikely and so a more appropriate level of 70-85% should be used to adequately plan for meeting supply needs.
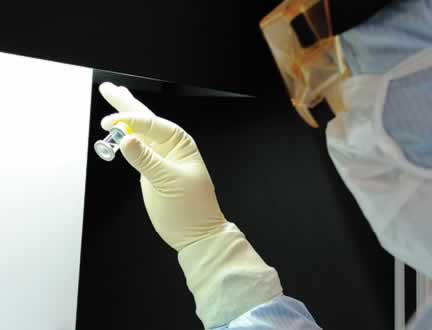
Because product demand and the number of patients treated will usually increase as a product progresses through clinical trial phases and on to commercialization, it is important that the designed process is scalable and that critical quality attributes are well characterized. Therefore, investing in process development and optimization must be appropriately timed, particularly if the product has been granted an accelerated approval process by regulators.
It is best to use consistent, scalable technologies throughout the development process when possible. For example, using a bioreactor system that is available in a range of sizes from the beginning allows for more predictable movement between each phase compared to a sudden switch in technology where significant process development may be necessary. Another example is the downstream purification of viral vectors. Many small-scale processes use cesium chloride ultracentrifugation, however, chromatographic methods are preferred for their scalability and better GMP control strategies. Attempting to switch to more scalable chromatographic methods half way through therapy development could significantly delay timelines and could potentially lead to a product that differs in purity from the original methodologies. Scalable processes also make it possible for cell therapy manufacturing to be produced at multiple GMP facilities rather than being restricted to a single site on a single piece of equipment.
It is also possible that a gene therapy will not need to be scaled-up, for instance in rare monogenic diseases with very low patient populations. In these cases it may be possible to meet all product demands without the need to scale-up. This is why understanding the scale of a disease indication is so critical in manufacturing process design.
In-house or Outsourced Manufacturing
The patient population being treated is a key consideration in deciding between in-house manufacturing versus outsourced manufacturing to a Contract Development and Manufacturing Organization (CDMO). In conventional medicine, the ratio of the incidence to prevalence of patients for a given disease is expected to be high so that capital expenditures, such as those required to build a manufacturing facility, can be amortized over a number of years. In contrast, for genetic diseases, the ratio of incidence to prevalence population can be quite low. This means that the prevalence population gets treated in the first few years after launch and then a manufacturing facility is only required to treat the incidence population which can be very small for rare and ultra-rare diseases. This means the capital associated with building a manufacturing facility for a single gene therapy has to amortized over just a couple of years after product launch. This can make the business case for building your own facility quite high.
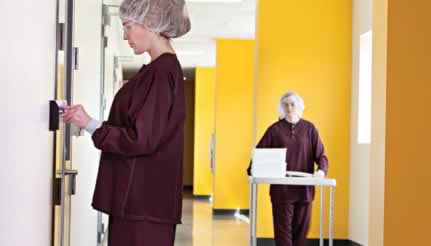
Beyond the expense and dedication of capital to build manufacturing capacity, time and resources required of gene therapy companies and their management must be considered. Companies must be able to establish the design and scale requirements for the facility, often with limited certainty as to the scale ultimately required, the potential launch quantities, and market penetration to guide planning. Outsourcing can serve as an effective and economical bridge until greater certainty is gained as to product demand.
However, for gene therapy companies who either have difficulty finding a CDMO partner or securing production slots in the timeframe they need, building a small, early phase GMP facility may make sense. There are also unique partnership agreements that can be established in lieu of full outsourcing, for example, a monoplant or condo arrangement.
Ultimately gene therapy companies will have to weigh several factors before deciding whether to outsource manufacturing or manufacture in-house and the appropriate time to implement each option.
Working with CDMOs and Suppliers to Ensure Technology Transfer Best Practices
A well-documented process, detailed bill of materials, and qualified analytical methods contribute to efficient technology transfer, as does the availability of subject matter experts with process knowledge at the targeted scale of manufacture.
When seeking to outsource production, contract negotiation and production slot reservation are first steps. Once technology transfer begins, developers of gene therapies need to consider that timeline from initiation of an order to the batch release of GMP vectors is the aggregate sum of time required for acquisition of plasmid DNA for transfection, of qualified raw materials and components, availability of manufacturing capacity and quality testing and final release of the vector. Allowing adequate time for each of these components requires effective planning, as does care in selection of available, GMP grade raw materials. Product specific analytical assays must also be ready for transfer as the lack of these tools can significantly hamper the progress toward GMP manufacturing.
Early interaction with a CDMO can be valuable and can establish realistic timelines for development and GMP manufacturing. The timeline from contract negotiation to released product can take up to 12 months. Often gaps that present hurdles to manufacturing can be identified and addressed in these early interactions. Moreover, adaption of the CDMO’s manufacturing platform can be leveraged to reduce the overall time and expenditures in creating their own process. For example, platforms established at CDMOs for upstream and downstream processing have already addressed availability of materials and components of appropriate quality for use in GMP manufacturing, so these common gaps are eliminated. Early interactions are also important in creating the custom and semi-custom assays used for in-process and release testing.
It is also important to engage with equipment providers early during the process. The experience of equipment providers for similar processes can be leveraged to facilitate design development.This can be done either onsite or as a contract service provided by the supplier if there are particular specialty pieces of equipment that you want to adopt into your process. In other words, aligning the equipment used in process development and analytical development with the equipment to be used in GMP manufacturing is an often overlooked, but critical aspect of having a successful technology transfer.
Logistics and Distribution
This is particularly important for cell-based gene therapies, which are personalized, autologous treatments. In these therapies, the coordination of collecting material from patients and shipping this material to and from the manufacturing area in a timely manner is critical. For instance Novartis has stated that their target manufacturing turnaround time from receipt of patient material to return of product is only 22 days. Gilead has stated that the median turnaround time for Yescarta® is 17 days. This kind of expedited turnaround on personalized therapies requires extensive data and temperature tracking systems to ensure a patient receives their own cells and also to ensure that the product is kept at the correct temperature.
Regulatory Approval
The challenge and benefit of the Breakthrough Therapy designation, often granted to gene therapy products, is the difference in the program development timeline compared to traditional biologics. Authors from the Friends of Cancer Research organization have published an article stating that median approval times for drugs with a breakthrough therapy designation is 2.9 months faster than traditional products. It is estimated that non-breakthrough therapy products are approved within 7.4 years from submission of the IND compared to 5.2 years for breakthrough therapy products.4
A successful gene therapy regulatory strategy requires: new product and process validation strategies to accommodate accelerated development timelines, active communication with the FDA and the dedication to pursuing and adopting new technologies to tackle vexing scientific challenges, particularly in the area of product characterization.
Typical gene therapy information requests that arise during reviews include providing more information on manufacturing methods and reagents used for master cell banks, working cell banks and the vector, information on plasmid manufacturing and release testing, freezing rates of the drug product, labeling methods for the frozen product, and method of assigning the product’s date of manufacture (see 21 CFR 610.50). As would be anticipated, there are many questions regarding analytical methods (e.g. potency), specifications and method validation. Specifications are typically based on a limited number of early phase lots, which leads to questions regarding comparability with the proposed commercial process. Similar to PEGylated biologics and the amount of free-PEG (Polyethylene glycol) in the product, regulators are also concerned with empty capsids controls.
As is often the case with emerging therapies, there are more questions than answers. However, gene therapies are unquestionably moving toward fulfilling their tremendous promise. Industry and regulators alike are working in an increasingly focused manner to determine how the needed regulatory constructs can be practically applied with product characterization technologies and better understanding of mechanics of action at the heart of the matter.
Reimbursement and Market Access
There are several factors to establishing a reimbursement strategy for gene therapies. One of the first is to keep the cost as reasonable as possible, recognizing that payers have limits, even if the therapies are one-time administration, life saving medications. Payers will be evaluating not only the efficacy and safety of a product but also its cost-effectiveness. Gene therapy companies need to engage early in the process with payers to ensure that their product will be covered.
Another important aspect is working with payers in advance to determine what documentation and data will be required to obtain reimbursement and broad coverage for patients. If the gene therapy has received an accelerated approval designation, this will reduce the time available to work with payers, so this will need to be done as quickly as possible.
In addition, coding needs to be considered. In order to receive payment in the United States, the gene therapy needs to have a code for reimbursement, which takes a significant amount of time to apply for and be granted. In fact, some novel products end up going to commercial launch without a billing code, which can delay patient access and payment. In cell-based gene therapies there are several components to treatment that all need coding, for instance cell collection, cell processing, and infusion back into the patient.
Because gene therapies are novel, payers will need education around the benefits of this type of therapy both clinically and from a long-term cost benefit perspective. Education is also critical in the area of cell-based gene therapy coding so that payers understand how the different components fit together and how they should be coded.
One gene therapy product that struggled with reimbursement and ultimately failed was Glybera®. Glybera® was granted EMA market authorization in 2012 to UniQure as an AAV gene therapy for lipoprotein lipase deficiency disease for which the patient population is very small. Treatment was a one-time series of injections of the viral vector carrying the intact copy of the lipoprotein lipase gene and the cost was around $1 million based on patient weight. Since approval in 2012, it has only been used and reimbursed once. Because Glybera® was never able to get approval from the national regulators in Europe, who decide which drugs get reimbursed, this left insurers to make decisions about whether treatment would be covered on a case-by-case basis and the burden of getting approval on the physician and patient.3,5 Due to the lack of use, UniQure will not submit renewal for marketing authorization of Glybera® from the European authorities when it expires in October.
In contrast, Spark Therapeutics has recognized that reimbursement is a major component of a gene therapies’ success. For their new therapy, Luxturna®, which corrects a rare inherited retinal disease, they have developed a novel threefold plan for ensuring coverage for their patients. The current cost of Luxturna®, is $850,000 per patient. In order to ensure reimbursement from payers, they are working to create contracts with individual payers or their specialty pharmacies who will then negotiate payment to the treatment centers to reduce the risk of non payment to the centers who decide to administer the therapy. They have also announced a rebate program based on treatment effectiveness at 30 to 90 days and then again at 30 months. If efficacy isn’t proven over those timeframes rebates will be provided to payers. Lastly, they are in discussions with the Centers for Medicare and Medicade Services to create a plan that would allow payers to reimburse the cost of the therapy in installments over several years.6
These innovative approaches for reimbursement of the more expensive gene therapies are a great step in the right direction of ensuring access to treatment for patients and the long term success of the therapy.
Provider and Patient Education and Access
It is important that providers and patients have access to the product. This access can be in the form of logistics, for example with cell-based gene therapies, making sure patients can get to a site to collect their cells or tissue and provide infusion of the treatment. It is important that providers understand proper collection and infusion methods. Cost is also a part of access, so securing reimbursement coverage is important. In addition, patients are increasingly becoming advocates of their own treatment and connection with thought leaders and patient advocacy groups can be invaluable in helping to educate the community on the product, as well as working with payers to ensure coverage. Having a commercial group involved early in a company’s evolution can be very beneficial. These professionals can make commercial projections, identify and interact with patient advocacy groups, and establish the framework for a suitable Target Product Profile. Initial and ongoing stakeholder education is a critical part of any commercialization plan.
In Closing
The past decade has seen a surge in the development of cell and gene therapies and recent successes have begun a paradigm shift in how we treat disease. The approval of these products will drive other similar products through the pipeline at an even faster pace because now there is a regulatory and manufacturing blueprint that industry stakeholders will be able to follow. However, it is important to remember that cell and gene therapies are still relatively new and there are still challenges regarding manufacturing and commercialization that need to be addressed.
The industry has a great appreciation for the challenges associated with manufacturing scale up and are actively looking to develop processes that are suitable for large-scale production. Previously, most manufacturing tools for cell and gene therapy were borrowed from either academic or from monoclonal antibody manufacturing and were often not fit for purpose. While this is still true of many tools, there are increasing numbers of tools that are designed specifically for viral vector manufacture and tools companies are now starting to invest in this. This is all good news in terms of creating manufacturing that is more efficient and cost-effective. These manufacturing improvements will enable more therapies that previously weren’t able to be manufactured efficiently or at a reasonable cost and will increase access and reimbursement of gene therapies as a whole.
This article is the first in a series that was published in our eBook, “Key Considerations for Gene Therapy Manufacturing for Commercialization”. You can download all the articles in the series, by downloading the eBook below.
Footnotes
-
1. Ginn, S.L., et al., Gene therapy clinical trials worldwide to 2017: An update. J Gene Med, 2018. 20(5): p. 3015.
-
2. Source: Alliance for Regenerative Medicine. State of the industry, 2018.
-
3. Roots Analysis Report: Gene therapy market 2015-2025, 2015.
-
4. raps.org. Analysis: breakthrough therapies cut development timeline by two years. 2016; Available from: www.raps.org/regulatory-focus/news-articles/2016/3/analysis-breakthrough-therapies-cut-development-timeline-by-two-years.
-
5. Regalado, A. The world’s most expensive medicine is a bust. 2016; Available from: www.technologyreview.com/s/601165/the-worlds-most-expensive-medicine-is-a-bust/.
-
6. Spark therapeutics announcesfirst-of-their-kind programs to improve patient access to LUXTURNA™ (voretigene neparvovec-rzyl), a one-time gene therapy treatment; Available from: ir.sparktx.com/news-releases/news-release-details/spark-therapeutics-announces-first-their-kind-programs-improve